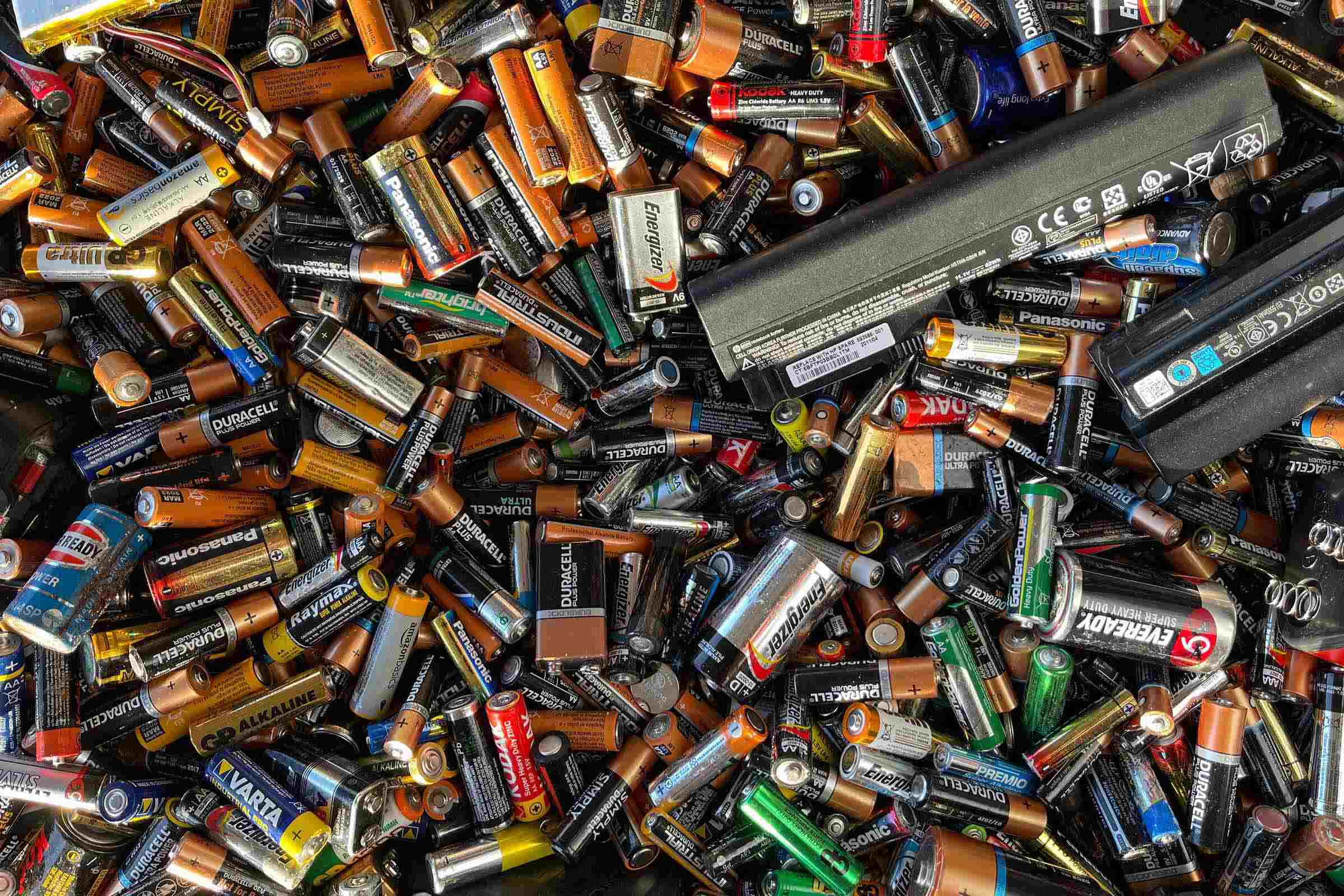
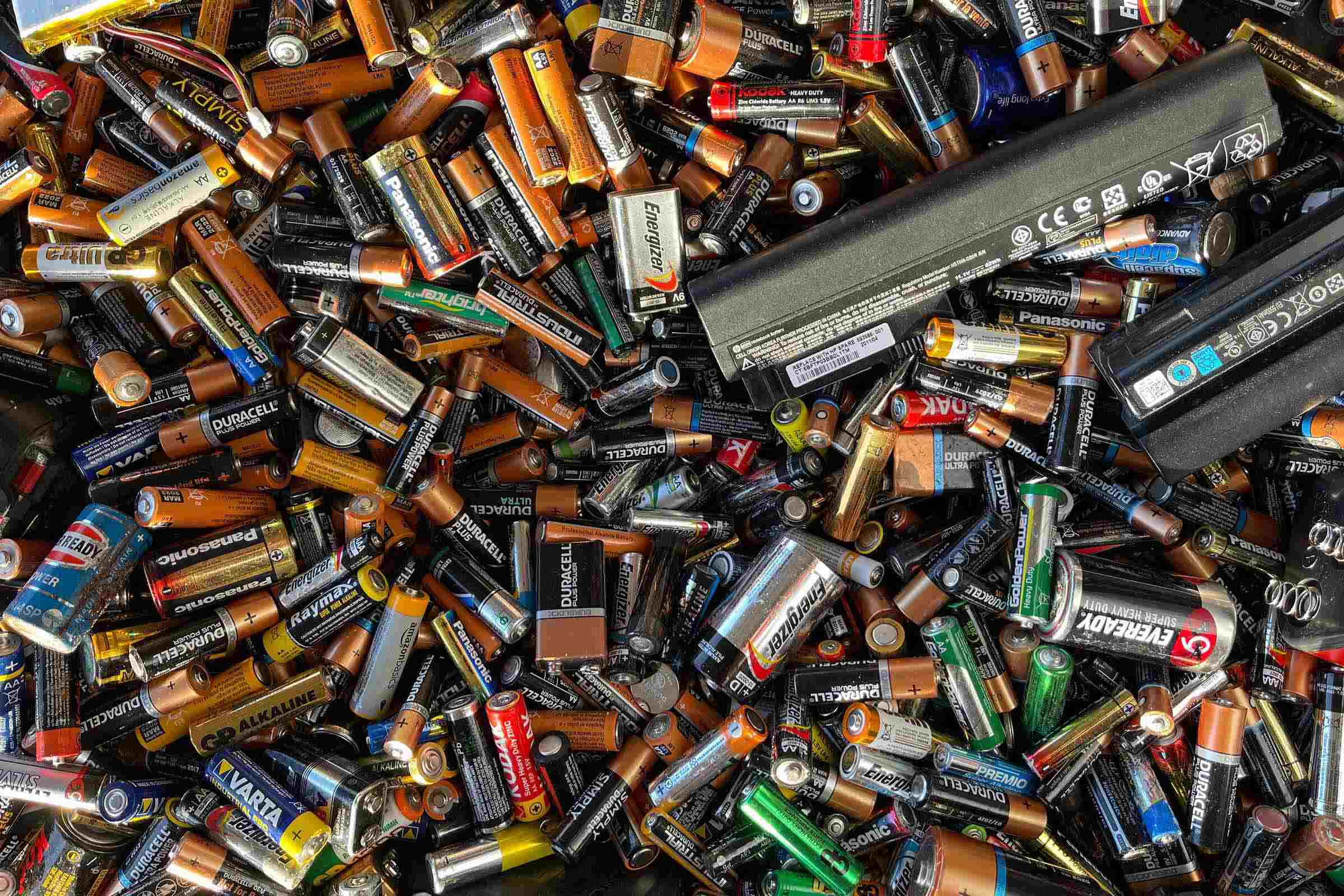
Articles
How To Store Energy In A Battery
Modified: February 23, 2024
Learn how to store energy in a battery with these informative articles. Discover tips, techniques, and important considerations for efficient energy storage.
(Many of the links in this article redirect to a specific reviewed product. Your purchase of these products through affiliate links helps to generate commission for Storables.com, at no extra cost. Learn more)
Introduction
Energy storage is a vital component of modern society, enabling us to store and utilize power efficiently. One of the most common and effective methods of energy storage is through the use of batteries. Batteries play a significant role in various applications, from powering electronic devices to storing renewable energy generated from solar panels or wind turbines.
In this article, we will explore the basic principles of battery energy storage and delve into the various types of batteries used for this purpose. We will also discuss the charging and discharging processes of batteries, factors affecting energy storage efficiency, the importance of battery management systems, advancements in battery technologies, and the environmental impact of battery energy storage. Furthermore, we will examine the wide range of applications for battery energy storage systems and the challenges and future directions in this field.
Battery energy storage is revolutionizing the way we store and utilize power, providing a sustainable and reliable solution for managing energy demands. With the global shift towards renewable energy sources, such as solar and wind, the need for efficient energy storage systems has become increasingly crucial.
Traditionally, electricity has been generated and consumed instantaneously. However, renewable energy sources have variable and intermittent output, making it necessary to store excess energy during periods of low demand and utilize it during times of high demand. This is where battery energy storage systems come into play, allowing us to store surplus energy efficiently and draw upon it when needed.
Furthermore, battery energy storage systems offer several advantages over other forms of energy storage, such as pumped hydro or compressed air. Batteries are compact, portable, and can be easily scaled up or down depending on the energy storage requirements. They also have a rapid response time, making them ideal for applications where quick bursts of power are needed, such as electric vehicles or grid stabilization.
As the demand for clean and sustainable energy solutions continues to grow, battery energy storage is becoming an integral part of our energy infrastructure. Proper understanding of battery technology and its applications is crucial for maximizing the benefits of energy storage and ensuring a smooth transition towards a greener and more sustainable future.
Key Takeaways:
- Battery energy storage systems are revolutionizing the energy landscape, offering solutions for renewable integration, grid stability, and off-grid power. Advancements in technology and sustainable practices are crucial for maximizing their potential.
- Addressing challenges such as cost reduction, environmental impact, and grid integration will drive the widespread adoption of battery energy storage. Embracing innovation and scalability is key to a sustainable energy future.
Read more: How To Store A Battery
Basic Principles of Battery Energy Storage
Before diving into the intricacies of battery energy storage, it’s important to understand the basic principles behind it. At its core, battery energy storage involves the conversion of electrical energy into chemical potential energy, which can be stored and later converted back into electrical energy when needed.
Batteries consist of one or more cells, each containing two electrodes – a positive electrode (cathode) and a negative electrode (anode). These electrodes are immersed in an electrolyte solution, creating a medium for the electrochemical reaction to occur.
When a battery is charged, a process known as oxidation occurs at the negative electrode. This involves the release of electrons, which travel through an external circuit to the positive electrode. Meanwhile, an opposite reaction known as reduction takes place at the positive electrode, where electrons combine with positive ions in the electrolyte solution.
During discharge, the opposite happens. The stored chemical potential energy is converted back into electrical energy as the chemical reactions are reversed. Electrons flow from the negative electrode through the external circuit to the positive electrode, generating an electric current that can be utilized to power devices or systems.
The voltage of a battery is determined by the difference in chemical potential between the positive and negative electrodes. This potential difference, also known as the cell voltage, is specific to the type of battery and the materials used in its construction.
It’s worth mentioning that different types of batteries have varying electrochemical reactions and mechanisms. For example, lead-acid batteries, commonly used in automotive applications, employ the reaction between lead oxide and lead to store and release energy. Lithium-ion batteries, on the other hand, utilize the movement of lithium ions between electrodes for energy storage.
Overall, battery energy storage relies on the principles of electrochemical reactions and the transformation of electrical energy into chemical potential energy. Understanding these principles is crucial for optimizing battery performance, improving efficiency, and ensuring the reliable storage and utilization of energy.
Types of Batteries Used for Energy Storage
There are several types of batteries used for energy storage, each with its own unique characteristics and applications. The choice of battery depends on factors such as energy storage capacity, power output, lifespan, and cost. Let’s explore some of the most commonly used battery technologies for energy storage:
- Lithium-ion (Li-ion) Batteries: Lithium-ion batteries have gained popularity in recent years due to their high energy density and long cycle life. These batteries utilize lithium ions that move between the positive and negative electrodes during charging and discharging. They are commonly used in portable electronics, electric vehicles, and grid-scale energy storage systems.
- Lead-Acid Batteries: Lead-acid batteries have been in use for over a century and are well-known for their reliability and low cost. These batteries utilize a chemical reaction between lead plates and sulfuric acid to store and release energy. They are commonly used in automotive applications, off-grid renewable energy systems, and backup power supply.
- Nickel-Cadmium (NiCd) Batteries: Nickel-cadmium batteries have been widely used for decades, especially in applications requiring high discharge rates. These batteries use nickel oxide hydroxide and metallic cadmium electrodes with an alkaline electrolyte. They are commonly found in emergency lighting, cordless power tools, and portable electronic devices.
- Nickel-Metal Hydride (NiMH) Batteries: Nickel-metal hydride batteries are an improvement over nickel-cadmium batteries, offering better energy density and reduced environmental impact. They use a hydrogen-absorbing metal alloy as the negative electrode, nickel oxide hydroxide as the positive electrode, and an alkaline electrolyte. NiMH batteries are commonly used in hybrid vehicles, portable electronic devices, and medical equipment.
- Sodium-Ion Batteries: Sodium-ion batteries are an emerging technology that shows promise for large-scale energy storage applications. These batteries use sodium ions instead of lithium ions for energy storage. They offer high energy density, low cost, and abundant raw materials. Sodium-ion batteries are being explored for use in renewable energy integration and grid-scale energy storage.
Each type of battery has its own advantages and limitations. Factors such as energy density, power density, cycle life, self-discharge rate, and environmental impact should be considered when selecting a battery for a specific energy storage application.
It’s worth noting that research and development in battery technology are ongoing, with advancements being made to improve performance, increase energy storage capacity, and reduce costs. As we continue to pursue cleaner and more efficient energy storage solutions, the evolution of battery technologies will play a crucial role in shaping the future of energy storage.
Charging and Discharging Process of Batteries
The charging and discharging process of batteries is a crucial aspect of energy storage. Understanding how batteries charge and discharge is essential for maximizing their efficiency and ensuring their longevity. Let’s take a closer look at the charging and discharging process:
Charging: When a battery is charged, a flow of electrical current is applied to it, causing a chemical reaction that stores energy. The charging process is typically divided into three stages: bulk charging, absorption charging, and float charging.
In the bulk charging stage, a constant current is applied to the battery, allowing it to quickly reach a high state of charge. During this stage, the battery voltage gradually increases while the battery absorbs the energy. Once the battery reaches a specific voltage threshold, it transitions to the absorption charging stage.
In the absorption charging stage, the battery voltage is held constant while the current gradually decreases. This stage allows the battery to fully charge and equilibrate, ensuring that all the chemical reactions within the battery are completed. Once the battery reaches its maximum capacity, it transitions to the float charging stage.
In the float charging stage, a lower constant voltage is applied to the battery to maintain its charge without overcharging it. This stage helps to prevent battery degradation and extends its lifespan. Float charging is commonly used in applications where batteries need to be constantly maintained at full charge, such as standby power systems or emergency backup systems.
Discharging: When a battery is discharged, the stored chemical energy is converted back into electrical energy, which can be used to power devices or systems. The discharging process occurs when a load is connected to the battery, creating a circuit for the flow of electrons.
During discharging, the electrons flow from the negative electrode (anode) to the positive electrode (cathode) through the external circuit, creating a current. Simultaneously, ions within the battery move from the negative electrode to the positive electrode through the electrolyte. This movement of electrons and ions allows for the release of stored energy and the generation of electrical power.
The rate at which a battery is discharged, also known as the discharge rate, affects its performance and capacity. Higher discharge rates can lead to a decrease in the overall capacity of the battery, while lower discharge rates can prolong its runtime.
It’s important to note that both the charging and discharging process can generate heat within the battery. This heat generation is influenced by factors such as the battery’s internal resistance, the rate of energy transfer, and the ambient temperature. Proper monitoring and management of battery temperature are critical to ensure safe and efficient operation.
Understanding the charging and discharging process of batteries is crucial for optimizing their performance, extending their lifespan, and ensuring reliable energy storage. It is important to follow recommended charging and discharging guidelines provided by the battery manufacturer to maintain the health and efficiency of the battery system.
Factors Affecting Battery Energy Storage Efficiency
The efficiency of battery energy storage systems is influenced by various factors that can impact their performance, duration, and overall effectiveness. Understanding these factors is crucial for optimizing the efficiency and maximizing the benefits of energy storage. Let’s explore some of the key factors that affect battery energy storage efficiency:
- Battery Type: Different battery chemistries have varying levels of efficiency. For example, lithium-ion batteries are known for their high energy density and efficiency, while lead-acid batteries have lower energy density and slightly lower efficiency. Choosing the right battery chemistry for the specific energy storage application is important to achieve optimal efficiency.
- Cycle Life: The cycle life of a battery refers to the number of charge and discharge cycles it can undergo before its capacity and performance degrade. Batteries with a higher cycle life tend to have better long-term efficiency, as they can maintain their performance over a longer period of time without significant degradation.
- Charging and Discharging Rates: The rate at which a battery is charged or discharged can impact its efficiency. High charging or discharging rates can lead to higher heat generation, which can reduce the overall efficiency of the battery. It is important to balance the desired power output with the limitations and specifications of the battery to achieve optimal efficiency.
- Temperature: Temperature has a significant effect on the performance and efficiency of batteries. Extreme temperatures, both hot and cold, can negatively impact battery efficiency and capacity. Operating batteries within their recommended temperature range helps maintain their efficiency and prolong their lifespan.
- Battery Management System (BMS): A well-designed and properly functioning BMS is essential for maximizing the efficiency of battery energy storage systems. A BMS monitors and controls various aspects of battery operation, including charging, discharging, temperature, and cell balancing. It helps optimize the charging and discharging process, prevents overcharging or overdischarging, and ensures balanced utilization of the battery cells.
- Depth of Discharge (DOD): The depth of discharge refers to the amount of battery capacity that is utilized during a discharge cycle. Higher DODs can lead to reduced battery life and lower overall efficiency. Limiting the depth of discharge and operating within recommended discharge ranges can help improve battery efficiency and prolong its lifespan.
- Aging and Degradation: Over time, batteries undergo natural aging and degradation, which can impact their efficiency and capacity. Factors such as calendar aging, cycle aging, and storage conditions can contribute to the degradation of battery performance. Periodic maintenance, proper storage, and regular performance assessments can help mitigate the effects of aging and degradation.
It’s important to note that the efficiency of battery energy storage systems is not solely determined by a single factor but rather by a combination of multiple factors. Optimal efficiency can be achieved by considering all these factors and selecting the right battery chemistry, implementing proper battery management systems, monitoring and controlling charging and discharging rates, and maintaining suitable operating conditions.
As battery technology continues to evolve, advancements in efficiency are being made to optimize energy storage systems and improve overall performance. By addressing and understanding the factors that affect battery energy storage efficiency, we can harness the full potential of battery technology and unlock its benefits for a wide range of applications.
Read more: How To Store Batteries
Importance of Battery Management Systems
Battery management systems (BMS) play a vital role in ensuring the safe, efficient, and reliable operation of battery energy storage systems. A BMS acts as the brain of the battery system, monitoring and controlling various parameters to optimize performance, prolong battery life, and enhance overall efficiency. Let’s explore the importance of BMS in battery energy storage:
- Safety: One of the primary functions of a BMS is to ensure the safety of the battery system. It continuously monitors and regulates parameters such as temperature, voltage, and current, preventing overcharging, overdischarging, and overheating. By maintaining safe operating conditions, a BMS helps mitigate the risk of thermal runaway, short circuits, and other safety hazards.
- Optimal Charging and Discharging: A BMS optimizes the charging and discharging process of batteries, ensuring that they operate within their recommended voltage and current ranges. It controls the charging algorithm to prevent overcharging, which can lead to battery damage and reduced efficiency. Similarly, it regulates the discharging process to prevent overdischarging, which can cause irreversible capacity loss.
- Battery Monitoring and Balancing: BMS continuously monitors the individual cells within a battery pack, ensuring that each cell operates at a similar voltage and capacity. It detects any cell imbalances and employs cell balancing techniques to equalize the charge across cells, improving overall battery performance and extending the lifespan of the battery.
- State of Charge (SOC) and State of Health (SOH) Monitoring: BMS accurately measures the state of charge and state of health of the battery. It provides real-time information about the available capacity, allowing users to effectively manage energy storage and optimize system performance. By monitoring the health of the battery, a BMS enables proactive maintenance and prevents premature failure.
- Efficiency Optimization: BMS helps maximize the efficiency of battery energy storage systems. It employs energy management techniques to minimize power losses during charging and discharging cycles. By ensuring that the battery operates within its optimal efficiency range, a BMS helps reduce energy waste and increases overall system efficiency.
- Data Logging and Analysis: A BMS collects and records data related to battery performance, including voltage, current, temperature, and charging/discharging cycles. This data can be analyzed to identify usage patterns, detect abnormalities, and optimize system performance. It provides valuable insights for system diagnostics, maintenance planning, and future battery performance improvements.
- Prognostics and Predictive Maintenance: BMS plays a crucial role in predicting and preventing battery failures. By analyzing historical data and monitoring key parameters, it can detect early signs of degradation or anomalies in battery performance. This allows for proactive maintenance and replacement of faulty components, minimizing downtime and ensuring continuous operation.
With the increasing importance of battery energy storage in various applications, the role of a BMS becomes even more critical. A well-designed and properly functioning BMS enhances the safety, efficiency, and reliability of battery energy storage systems, enabling optimal utilization of energy resources and contributing to a sustainable and resilient energy infrastructure.
As battery technology continues to evolve, advancements in BMS are being made to improve accuracy, expand functionality, and enhance system integration. By leveraging the capabilities of BMS, we can unlock the full potential of battery energy storage and accelerate the adoption of clean and efficient energy solutions.
When storing energy in a battery, make sure to keep it at a moderate temperature, avoid overcharging, and store it in a dry, cool place to prolong its lifespan.
Advancements in Battery Technologies for Energy Storage
Battery technologies continue to advance at a rapid pace, driven by the increasing demand for energy storage solutions that are more efficient, reliable, and sustainable. These advancements are crucial in meeting the growing needs of renewable energy integration, electrification of transportation, and grid stability. Let’s explore some of the key advancements in battery technologies for energy storage:
- Lithium-ion (Li-ion) Battery Technology: Li-ion batteries have dominated the market and witnessed significant advancements over the years. Innovations in cathode and anode materials, electrolyte composition, and cell design have led to improved energy density, longer cycle life, and enhanced safety. These advancements have made Li-ion batteries the go-to choice for various energy storage applications, from portable electronics to electric vehicles and grid-scale installations.
- Solid-State Batteries: Solid-state batteries are a promising next-generation technology that offers higher energy density, improved safety, and longer lifespan compared to traditional Li-ion batteries. Instead of using liquid electrolytes, solid-state batteries utilize solid electrolytes, eliminating the risk of leakage and thermal runaway. With ongoing research and development, solid-state batteries are expected to revolutionize energy storage by providing even greater energy density and faster charging capabilities.
- Lithium-Sulfur (Li-S) Batteries: Li-S batteries have gained attention as an alternative to Li-ion batteries due to their high energy density and lower cost. They utilize sulfur as the cathode material, which offers a much higher theoretical energy density than conventional cathode materials. However, challenges such as the “shuttle effect” and limited cycle life are being addressed through the development of advanced cathode designs and electrolyte optimization.
- Flow Batteries: Flow batteries are a unique approach to energy storage, utilizing electrochemical reactions between liquid electrolytes stored in external tanks. These batteries offer advantages such as flexible capacity scaling, long cycle life, and a decoupling of power and energy ratings. Recent advancements in flow battery technology include the development of new electrolyte chemistries, membrane materials, and cell designs, resulting in improved energy efficiency and cost-effectiveness.
- Sodium-Ion (Na-ion) Batteries: Sodium-ion batteries are being explored as a low-cost alternative to lithium-ion batteries, utilizing abundant sodium resources. Advancements in Na-ion battery technology aim to increase their energy density, cycle life, and overall performance. By overcoming challenges such as lower energy density and larger cell volume, Na-ion batteries have the potential to become a cost-effective solution for stationary energy storage.
- Advanced Battery Management Systems (BMS): Alongside advancements in battery chemistry, the development of advanced BMS has played a key role in optimizing battery performance. BMSs now incorporate sophisticated algorithms for state of charge estimation, cell balancing, thermal management, and predictive maintenance. These advancements enable more accurate monitoring, improved efficiency, and enhanced safety for battery energy storage systems.
- Recycling and Sustainability: As the adoption of battery energy storage increases, there is a growing focus on the recycling and sustainability of battery materials. Advancements in battery recycling technologies allow for efficient extraction of critical metals, such as lithium, cobalt, and nickel, reducing the environmental impact and ensuring the circular economy of battery materials.
These advancements in battery technologies are transforming the landscape of energy storage, making it more efficient, cost-effective, and environmentally friendly. As research and development efforts continue, we can expect to see further improvements in energy density, lifespan, safety, and cost, making energy storage an integral part of our transition towards a clean and sustainable energy future.
Environmental Impact of Battery Energy Storage
Battery energy storage systems have the potential to contribute significantly to a cleaner and more sustainable energy future. However, it is important to consider the environmental impact of these systems throughout their lifecycle. Let’s explore the various aspects of the environmental impact of battery energy storage:
- Raw Material Extraction: The production of batteries involves the extraction of raw materials such as lithium, cobalt, nickel, and other rare earth metals. The mining and processing of these materials can have negative environmental and social impacts. It is important to ensure responsible mining practices, promote recycling, and explore alternative materials to minimize the environmental footprint of raw material extraction.
- Manufacturing and Assembly: The manufacturing and assembly processes of batteries consume energy and generate waste. Optimizing these processes to reduce energy consumption, minimize emissions, and minimize waste generation is crucial. Efforts are being made to improve manufacturing techniques, increase production efficiency, and implement recycling programs to reduce the environmental impact of battery production.
- Energy Efficiency: Battery energy storage systems play a crucial role in improving the efficiency of energy utilization. By storing and releasing energy when it is needed most, batteries can help reduce energy waste and optimize power generation and consumption. This improved energy efficiency can lead to a reduction in greenhouse gas emissions and a more sustainable use of resources.
- Life Cycle Considerations: The environmental impact of battery energy storage should be evaluated throughout its entire life cycle, including transportation, installation, operation, and end-of-life management. Proper recycling and disposal of batteries are essential to prevent environmental contamination and minimize the impact of hazardous materials.
- Renewable Energy Integration: Battery energy storage systems are crucial for integrating renewable energy sources, such as solar and wind, into the grid. By storing excess energy during periods of high production and releasing it during periods of high demand, batteries help balance the grid and reduce the reliance on fossil fuel-based power generation. This contributes to a significant reduction in greenhouse gas emissions and promotes a cleaner and more sustainable energy mix.
- Recycling and Circular Economy: The recycling and reuse of batteries are important for reducing environmental impact and conserving valuable resources. Efforts are being made to develop efficient and environmentally friendly recycling technologies, allowing the recovery of valuable materials from used batteries. Implementing a circular economy approach ensures that batteries are recycled, and the extracted materials are used to manufacture new batteries, minimizing the need for raw material extraction.
- End-of-Life Management: Proper end-of-life management of batteries is crucial to prevent environmental contamination and ensure the safe disposal or recycling of used batteries. Establishing convenient and safe collection systems, promoting recycling programs, and implementing strict regulations for the disposal of batteries are essential to mitigate the environmental impact of battery waste.
The environmental impact of battery energy storage systems can be significant, but with proper management and responsible practices, the negative effects can be minimized. It is important for industry stakeholders, policymakers, and consumers to work together to promote sustainable battery production, increase recycling rates, and implement comprehensive environmental management strategies throughout the life cycle of batteries.
By prioritizing sustainability and adopting environmentally conscious practices, we can harness the benefits of battery energy storage while minimizing its impact on the environment, ultimately contributing to a more sustainable and resilient energy future.
Applications of Battery Energy Storage Systems
Battery energy storage systems have a wide range of applications in various industries, enabling efficient energy management and contributing to a more sustainable and reliable energy infrastructure. Let’s explore some of the key applications of battery energy storage systems:
- Renewable Integration: Battery energy storage systems play a crucial role in integrating renewable energy sources into the grid. They help address the intermittent nature of solar and wind power by storing excess energy during periods of high production and releasing it during periods of high demand. This not only ensures a stable and reliable power supply but also promotes the increased utilization of renewable energy resources.
- Microgrids and Island Systems: In remote areas or island communities, battery energy storage systems can provide energy independence and grid resilience. By storing excess energy from renewable sources, microgrids and island systems can reduce reliance on fossil fuels and ensure a reliable power supply when the main grid is unavailable or unreliable. This is particularly valuable during emergencies or natural disasters.
- Peak Shaving and Load Management: Battery energy storage systems are employed to manage peak demand and reduce energy costs for commercial and industrial consumers. By storing energy during periods of low demand and releasing it during peak demand, batteries help alleviate stress on the grid and optimize energy consumption, ultimately reducing electricity costs for consumers.
- Frequency Regulation and Grid Stabilization: Battery energy storage systems provide rapid response capabilities that support frequency regulation and grid stabilization. They can inject or absorb power within milliseconds, helping to maintain steady grid frequency and voltage levels. This is particularly important as the grid incorporates higher levels of distributed energy resources and intermittent renewable generation.
- Electric Vehicle (EV) Charging Infrastructure: Battery energy storage systems are used to support fast-charging infrastructure for electric vehicles. By storing excess energy during off-peak hours and releasing it during peak charging periods, batteries can alleviate stress on the main grid and ensure reliable and efficient charging for EVs. This helps accelerate the adoption of electric vehicles and contributes to the reduction of greenhouse gas emissions in the transportation sector.
- Uninterruptible Power Supply (UPS) Systems: Battery energy storage systems are crucial for providing backup power during power outages and ensuring uninterrupted operation of critical systems and equipment. UPS systems equipped with batteries can act as a reliable power source, offering seamless transitions from grid power to battery power, and allowing time for backup generators to start up, if necessary.
- Off-Grid and Remote Power Systems: In areas without access to reliable electricity grids, battery energy storage provides a viable solution for off-grid power systems. Batteries store energy generated from renewable sources or other power generation methods, such as diesel generators or small-scale hydroelectric systems, and provide a consistent and reliable power supply to meet the needs of remote communities, telecommunications towers, and remote monitoring stations.
The applications of battery energy storage systems are diverse and continue to expand as technology advances and the need for sustainable and efficient energy solutions grows. Battery energy storage is transforming the way we generate, store, and utilize energy, enabling a more flexible, resilient, and sustainable energy infrastructure across various sectors.
As the demand for clean energy continues to increase, the versatility and scalability of battery energy storage systems make them a vital tool in the transition to a more sustainable future.
Read more: How To Store New Batteries
Challenges and Future Directions in Battery Energy Storage
Battery energy storage systems have made significant progress in recent years, but there are still challenges to overcome and areas for further improvement. As technology continues to advance and the demand for energy storage grows, the following challenges and future directions in battery energy storage are worth considering:
- Cost Reduction: One of the main challenges in battery energy storage is the cost. While the cost of batteries has decreased over time, further reductions are needed to improve affordability and accelerate widespread adoption. Continued advancements in manufacturing processes, economies of scale, and research and development efforts can help drive down costs and make energy storage systems more accessible to a wider range of applications and consumers.
- Energy Density: Increasing energy density is a critical focus for battery energy storage systems. Higher energy density means that more energy can be stored in a given volume or weight, enabling longer durations of energy storage and improved system performance. Advancements in battery chemistry and materials, along with research into new battery technologies, will contribute to achieving higher energy densities and improving overall system efficiency.
- Longevity and Cycle Life: Battery lifespan and cycle life are important factors in determining the economic and environmental viability of energy storage systems. Improving battery durability, increasing the number of charge-discharge cycles, and enhancing the ability to withstand extreme operating conditions will extend battery life, reduce the need for replacements, and improve the sustainability of energy storage deployments.
- Environmental Impact: While energy storage systems offer environmental benefits, it is essential to address the environmental impact of battery production, raw material extraction, and end-of-life management. Advancements in battery recycling technologies, the use of more sustainable materials, and the implementation of responsible manufacturing practices will help minimize the environmental footprint of battery energy storage systems.
- Integration and Grid Interoperability: Seamless integration of battery energy storage systems into existing energy grids presents technical and regulatory challenges. Efficient bidirectional power flow, grid compatibility, and standardization of interfaces and communication protocols are important for achieving seamless integration. Establishing clear regulatory frameworks and market incentives that support the deployment and operation of energy storage systems will facilitate grid interoperability and maximize the benefits of energy storage for the grid.
- Technological Innovations: Research and development efforts are driving innovations in battery technology, exploring new materials, alternative chemistries, and novel designs. The development of solid-state batteries, sodium-ion batteries, metal-air batteries, and other emerging technologies holds the promise of enhanced performance, improved safety, and greater sustainability in the future. Continued investment in research and collaboration between academia, industry, and government institutions will accelerate technological advancements in battery energy storage.
- Scalability and Flexibility: Battery energy storage systems need to be scalable and flexible to accommodate various applications and requirements. Standardization of battery form factors, interconnection methods, and communication protocols can help improve interoperability and facilitate scalability. Modular designs and flexible energy management systems will enable seamless integration and adaptability to different energy storage needs.
The future of battery energy storage is bright, with ongoing technological advancements, cost reductions, and policy support. As the industry continues to evolve, addressing these challenges and embracing future directions will contribute to the widespread adoption of battery energy storage, transforming the energy landscape and accelerating the transition to a more sustainable and resilient energy future.
Conclusion
Battery energy storage systems have emerged as a crucial component in our journey towards a cleaner, more sustainable, and efficient energy future. They offer numerous benefits, from integrating renewable energy sources and improving grid stability to providing backup power and optimizing energy utilization. However, there are challenges to overcome and areas for further development to maximize the potential of battery energy storage.
Advancements in battery technology, including lithium-ion, solid-state, and flow batteries, have significantly improved energy density, cycle life, and safety. These advancements have enabled the widespread adoption of battery energy storage systems across various industries and applications.
Battery management systems play a vital role in ensuring the safe, efficient, and reliable operation of battery energy storage. They monitor and control parameters such as charging, discharging, temperature, and cell balancing, optimizing performance and extending battery life.
While battery energy storage systems offer immense potential, there are challenges to address. Cost reduction, increasing energy density, improving battery longevity, managing environmental impact, integrating with existing grids, fostering technological innovations, achieving scalability and flexibility – these are some of the key challenges that industry stakeholders, policymakers, and researchers must work together to overcome.
Looking towards the future, the continued advancements in battery technology, coupled with policy support and market incentives, hold great promise. Reductions in cost, improvements in energy density, and increased environmental sustainability will drive the widespread adoption of battery energy storage systems.
The applications of battery energy storage are vast and evolving. From renewable energy integration and microgrids to peak shaving, EV charging infrastructure, and off-grid systems, battery energy storage plays a central role in enhancing energy efficiency, grid stability, and resilience.
In conclusion, battery energy storage systems are revolutionizing the way we generate, store, and utilize energy. By addressing the challenges, pursuing technological innovations, and embracing sustainable practices, we can fully unlock the potential of battery energy storage. This will pave the way for a greener, more reliable, and sustainable energy future, benefiting both present and future generations.
Frequently Asked Questions about How To Store Energy In A Battery
Was this page helpful?
At Storables.com, we guarantee accurate and reliable information. Our content, validated by Expert Board Contributors, is crafted following stringent Editorial Policies. We're committed to providing you with well-researched, expert-backed insights for all your informational needs.
0 thoughts on “How To Store Energy In A Battery”