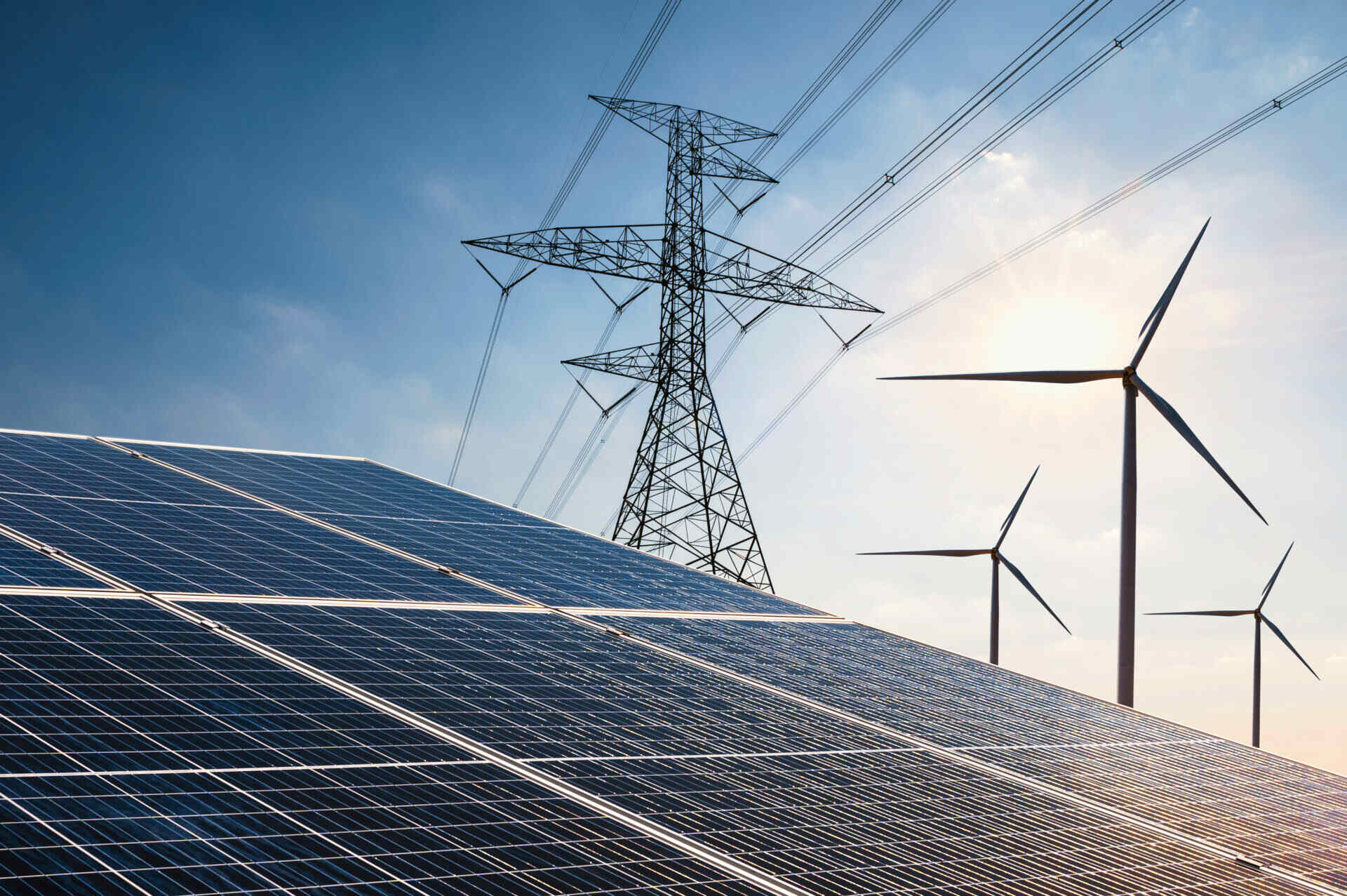
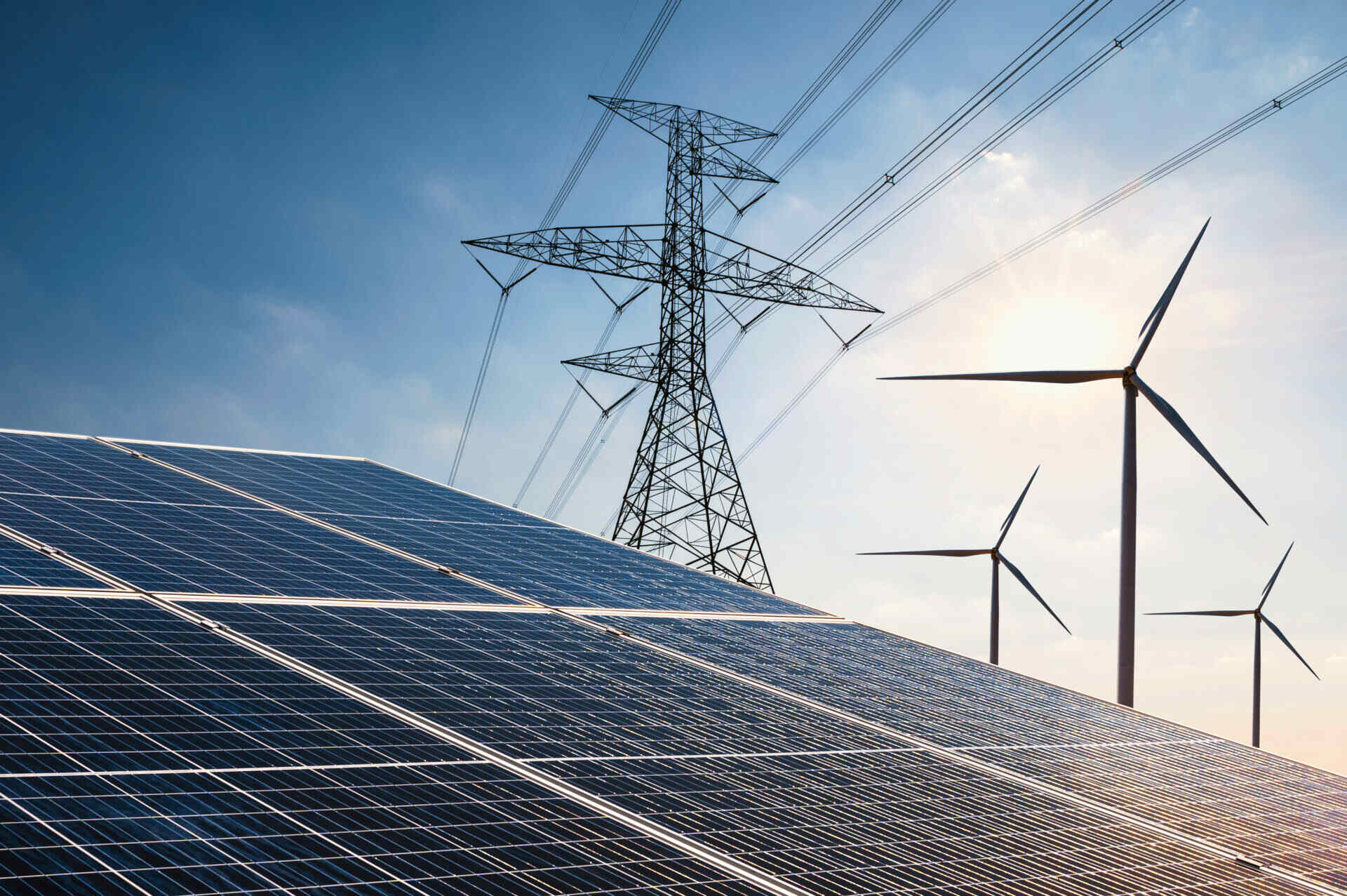
Articles
How To Store Energy
Modified: January 23, 2024
Discover the best articles on how to store energy and maximize efficiency. Learn innovative techniques and tips to save energy and reduce costs.
(Many of the links in this article redirect to a specific reviewed product. Your purchase of these products through affiliate links helps to generate commission for Storables.com, at no extra cost. Learn more)
Introduction
Energy storage plays a crucial role in our modern world, where the demand for reliable and sustainable energy solutions is growing exponentially. It provides the ability to store excess energy generated during periods of low demand or high production and utilize it during times of high demand or low production. The ability to efficiently store energy not only improves the stability and reliability of our energy grid but also enables the integration of renewable energy sources, such as solar and wind power, which are inherently intermittent.
Energy storage technologies have come a long way in recent years, presenting a wide range of options to store and deliver energy when and where it is needed. These advancements offer numerous benefits, including increased grid flexibility, reduced energy costs, improved efficiency, and reduced carbon emissions.
In this article, we will explore the various methods of energy storage, highlighting their benefits and limitations. We will also delve into the latest advancements in energy storage technologies and their potential future applications.
Key Takeaways:
- Energy storage technologies, including batteries, pumped hydro storage, compressed air energy storage, flywheels, and thermal energy storage, offer diverse solutions for grid stability, renewable energy integration, cost savings, and emergency preparedness.
- Advancements in energy storage technologies, such as lithium-ion batteries, flow batteries, solid-state batteries, hydrogen fuel cells, and advanced thermal energy storage, are driving the transition to a sustainable and decentralized energy system, despite challenges related to cost, technology maturity, and environmental impact.
Read more: How Does A Solar Water Heater Work
Benefits of Energy Storage
Energy storage systems offer several benefits that contribute to a more sustainable and efficient energy infrastructure. Let’s take a closer look at some of the key advantages:
- Grid Stability and Reliability: Energy storage helps maintain a stable and reliable grid by balancing the supply and demand of electricity. During periods of high demand, stored energy can be deployed to meet the load, reducing the strain on power generation facilities and minimizing the risk of blackouts or brownouts.
- Integration of Renewable Energy: One of the biggest challenges with renewable energy sources is their intermittent nature. Energy storage enables the capture and storage of excess energy generated from renewable sources during periods of high production, such as during sunny or windy days. This stored energy can then be released when demand exceeds the renewable energy generation, ensuring a consistent and reliable power supply.
- Peak Shaving and Load Management: Energy demand often fluctuates throughout the day, with peaks occurring during specific hours. By storing excess energy during periods of low demand, energy storage systems can release this stored energy during peak hours, reducing the strain on power grids and mitigating the need for additional power generation capacity.
- Cost Savings and Efficiency: Energy storage systems can enhance the efficiency of power grids by reducing line losses and optimizing energy flows. Additionally, these systems can help to lower electricity costs by storing energy during off-peak hours when electricity prices are lower and delivering it during peak hours when prices are higher.
- Backup Power and Emergency Preparedness: Energy storage systems can act as a reliable backup power source during emergencies or power outages. This is particularly beneficial in critical facilities such as hospitals, data centers, and telecommunications infrastructure where uninterrupted power supply is crucial.
The benefits of energy storage extend beyond the electrical grid. They also have applications in transportation, where electric vehicles can benefit from advanced battery technologies to store and utilize electricity efficiently, reducing reliance on fossil fuels and contributing to lower emissions.
Overall, energy storage technologies empower us to have more control over our energy consumption, foster grid stability, facilitate the integration of renewable energy, and pave the way for a more sustainable and resilient energy future.
Different Methods of Energy Storage
Energy storage technologies encompass a wide range of methods and systems. Here are some of the most common and promising methods of energy storage:
- Batteries: Batteries are perhaps the most well-known and widely used energy storage devices. They store energy in chemical form and convert it back to electrical energy when needed. Lithium-ion batteries, for example, are commonly used in portable electronics and electric vehicles (EVs) due to their high energy density and long cycle life. Other battery technologies, such as flow batteries and sodium-ion batteries, are also being developed and deployed for various applications.
- Pumped Hydro Storage: Pumped hydro storage involves the use of elevated reservoirs of water to store potential energy. During periods of low electricity demand, excess electricity is used to pump water from a lower reservoir to an upper reservoir. When electricity demand is high, the stored water is released back to the lower reservoir, passing through turbines to generate electricity. This method is highly efficient and currently accounts for the majority of grid-scale energy storage globally.
- Compressed Air Energy Storage (CAES): CAES systems store energy by compressing air and storing it in underground caverns or tanks. When electricity demand is high, the compressed air is released, expanded through turbines to generate electricity. Some CAES systems utilize the waste heat generated during compression to improve efficiency. CAES is known for its long-duration storage capabilities and has potential for large-scale energy storage applications.
- Flywheels: Flywheels store energy in the form of rotational kinetic energy. They consist of a spinning rotor inside a vacuum-sealed container that minimizes energy losses. When electricity demand increases, the kinetic energy is released and converted back to electricity through a generator. Flywheel energy storage systems are fast-responding and suitable for applications that require short-duration energy storage and rapid power delivery.
- Thermal Energy Storage: Thermal energy storage utilizes the storage and release of heat energy for various applications. One common method is the use of phase change materials (PCMs) that can store and release heat by changing their physical state, such as from solid to liquid or vice versa. Thermal energy storage finds applications in space heating and cooling, industrial processes, and solar thermal power plants.
These are just a few examples of the diverse and evolving methods of energy storage. Each method has its own advantages and limitations, and their suitability depends on factors such as scale, duration, response time, efficiency, and cost considerations.
It’s worth noting that ongoing research and development are leading to the emergence of new and innovative energy storage technologies, such as hydrogen fuel cells and advanced flow batteries. These advancements promise even greater energy storage capacity, efficiency, and sustainability in the future.
Batteries
Batteries are a widely recognized and extensively used form of energy storage. They convert chemical energy into electrical energy and are commonly utilized in portable electronics, electric vehicles (EVs), and grid-scale energy storage applications. Batteries play a crucial role in enabling the transition to a more sustainable and renewable energy future.
One of the most prevalent battery technologies is the lithium-ion battery, known for its high energy density, long cycle life, and low self-discharge rate. Lithium-ion batteries are commonly found in smartphones, laptops, and electric vehicles due to their ability to deliver a high amount of power in a compact and lightweight package. Their widespread adoption has been driven by advancements in electrode materials, such as lithium iron phosphate and lithium nickel cobalt aluminum oxide, which enhance their performance and safety.
Flow batteries have also gained attention in recent years. These batteries store energy in electrolyte solutions contained in separate tanks. During operation, the electrolytes are circulated through an electrochemical cell, causing a chemical reaction that produces electricity. The advantage of flow batteries is their scalability and long cycle life, making them suitable for stationary energy storage applications. Some notable examples of flow battery technologies include vanadium redox flow batteries and zinc-bromine flow batteries.
Other battery technologies are also being explored for specific applications. For example, sodium-ion batteries are being developed as a potential alternative to lithium-ion batteries due to the abundance and low cost of sodium. Solid-state batteries, which use a solid electrolyte instead of a liquid or gel electrolyte, show promise for higher energy densities, improved safety, and faster charging capabilities.
Advancements in battery technologies are focused on improving their capacity, efficiency, and safety while reducing their cost and environmental impact. Researchers and engineers are actively exploring the use of new materials, such as silicon anodes and solid-state electrolytes, to enhance battery performance. Additionally, efforts are being made to develop recycling and repurposing methods for batteries to minimize waste and maximize resource utilization.
The role of batteries in energy storage extends beyond portable electronics and transportation. They are increasingly being deployed in grid-scale applications to support renewable energy integration, improve grid stability, and balance supply and demand. By storing excess energy generated from renewable sources during times of low demand or high production, batteries can release that stored energy during peak demand periods, reducing the need for fossil fuel-based power generation and enhancing the reliability of the grid.
Despite their many advantages, batteries do have limitations. The production of battery materials can have environmental implications, and their disposal raises concerns about waste management. Furthermore, certain battery technologies may experience degradation and capacity loss over time, necessitating periodic replacement.
Nevertheless, the ongoing research and development in battery technologies hold immense potential for energy storage and contribute significantly to the transition towards a more sustainable and decentralized energy system.
Pumped Hydro Storage
Pumped hydro storage is one of the most well-established and widely used methods of energy storage, accounting for the majority of grid-scale storage capacity worldwide. It utilizes the stored potential energy of water to generate electricity when needed.
The concept of pumped hydro storage is relatively straightforward. During periods of low electricity demand or high availability of renewable energy, such as excess electricity from solar or wind power, water is pumped from a lower reservoir to a higher reservoir. This process typically occurs during off-peak hours when electricity prices are lower.
When electricity demand increases or renewable energy generation decreases, the stored water is released from the upper reservoir back to the lower reservoir, passing through turbines to generate electricity. The kinetic energy of the falling water is converted into electrical energy, which can be supplied to the grid.
Pumped hydro storage offers several advantages. First, it provides a large-scale energy storage solution with the ability to store and release gigawatt-hours of electricity. This makes it suitable for balancing the fluctuating supply and demand patterns of the electrical grid.
Additionally, pumped hydro storage has high round-trip efficiency, meaning that a significant portion of the stored energy is recovered when the water is released back to the lower reservoir. This makes it an efficient and reliable method of energy storage.
Pumped hydro storage also has a long lifespan and can operate for decades with minimal maintenance. The technology is well-proven and has been implemented successfully in various regions around the world for many years.
However, pumped hydro storage also has certain limitations and constraints. The availability of suitable geographic locations with the required topographical features, such as two reservoirs at different elevations and a sufficient water source, can present a challenge. Additionally, the construction of pumped hydro storage facilities can be capital-intensive and may face environmental considerations.
Despite these challenges, pumped hydro storage continues to play a crucial role in the energy storage landscape. It provides grid operators with a reliable and flexible tool to manage electricity supply and demand, integrate renewable energy sources, and ensure the stability and reliability of the electrical grid.
As the demand for energy storage grows and new technologies emerge, pumped hydro storage remains an essential component of our energy infrastructure, providing a tried-and-true solution for storing and delivering electricity when and where it is needed.
Read more: How Often Should A Refrigerator Run
Compressed Air Energy Storage (CAES)
Compressed Air Energy Storage (CAES) is a method of storing and releasing energy that utilizes compressed air to store potential energy for later use. It is a promising technology for large-scale, long-duration energy storage applications.
The CAES process involves two main stages: compression and expansion. During off-peak hours when electricity demand is low, excess electricity is used to power compressors that compress air and store it in underground caverns or aboveground tanks. The compressed air is stored at a high pressure, which serves as potential energy.
When electricity demand increases or when renewable energy generation is insufficient, the stored compressed air is released and expands through a turbine. As it expands, the air drives the turbine, which is connected to a generator, thereby converting the stored potential energy back into electricity. Additional heat can be supplied during the expansion process to increase the system’s efficiency.
CAES systems have several advantages. One key advantage is their potential for long-duration energy storage. Unlike some other energy storage methods that may have limited storage durations, CAES systems can store and release energy for several hours or even days. This makes them suitable for managing fluctuating electricity demand over extended periods, such as during seasonal variations or multi-day low renewable energy generation periods.
Furthermore, CAES systems have a relatively high round-trip efficiency, allowing for a significant amount of stored energy to be recovered during the expansion process. Efficiency can be further enhanced by utilizing waste heat generated during the compression process for other heat-dependent processes or by integrating the system with renewable energy sources.
Another advantage of CAES is its scalability. As the size and capacity of energy storage systems increase, CAES becomes an attractive option due to its ability to utilize existing infrastructure, such as natural gas pipelines, and its potential for utilizing abandoned gas or oil fields for underground air storage.
However, there are some challenges and considerations associated with CAES. In particular, the geological suitability for underground air storage caverns needs to be carefully assessed, as not all locations are suitable for their construction. Additionally, the compression and expansion processes can result in energy losses due to inefficiencies and heat dissipation.
Efforts are underway to address these challenges and improve the efficiency and cost-effectiveness of CAES systems. Advances in thermodynamic modeling, improved compressor and turbine designs, and the integration of renewable energy sources are helping to optimize and enhance the performance of CAES systems.
Compressed Air Energy Storage offers a promising solution for large-scale energy storage, enabling the integration of renewable energy, managing grid stability, and providing a reliable and flexible source of electricity. As technology continues to evolve and improve, CAES has the potential to play a significant role in our transition to a cleaner and more sustainable energy future.
Consider using rechargeable batteries or a power bank to store energy for small electronic devices. This can help you save money and reduce waste from disposable batteries.
Flywheels
Flywheel energy storage is a mechanical method of storing energy in the form of rotational kinetic energy. It involves spinning a rotor at high speeds and using the stored energy to generate electricity when needed. Flywheels have gained attention as a fast-responding and efficient solution for short-duration energy storage applications.
The basic principle of a flywheel energy storage system revolves around the conservation of angular momentum. The flywheel rotor is connected to a motor-generator that can both accelerate and decelerate the rotor. During periods of excess electricity or low demand, the motor-generators accelerate the flywheel, storing energy in the form of kinetic energy.
When electricity demand increases or when renewable energy generation decreases, the kinetic energy of the spinning rotor is converted back into electrical energy through the motor-generators. This process allows for the efficient release of stored energy and rapid power delivery.
Flywheel energy storage systems offer several advantages. First and foremost, their rapid response time makes them ideal for applications that require immediate power support or quick frequency regulation. They can respond within milliseconds, helping to stabilize the electrical grid during sudden fluctuations in supply or demand.
Additionally, flywheels have a high round-trip efficiency, meaning that a significant portion of the stored energy is recovered during discharge. Their mechanical nature allows for minimal energy losses and faster charge/discharge cycles compared to some other energy storage technologies.
Flywheels also have a long lifespan with little degradation over time. The mechanical components are designed for durability and reliability, allowing for frequent charge and discharge cycles without significant performance degradation.
However, flywheel energy storage systems do have limitations. One of the challenges is the storage duration, as the energy stored in a flywheel can be quickly released. This makes flywheels better suited for short-duration energy storage applications rather than long-term energy storage needs.
Another consideration for flywheel systems is their physical size and weight. The flywheel rotor needs to be carefully designed and constructed to withstand high rotational speeds, which can lead to larger and heavier systems compared to some other energy storage technologies.
Despite these limitations, flywheel energy storage continues to evolve and find niche applications. Research and development efforts are focused on improving design efficiency, reducing system costs, and exploring innovative materials, such as carbon fiber composites, to further enhance performance.
Overall, flywheel energy storage systems offer a unique combination of fast response times, high efficiency, and durability. As their technology continues to advance, they hold promise for supporting grid stability, providing backup power, and enabling the integration of renewable energy sources into our energy infrastructure.
Thermal Energy Storage
Thermal energy storage (TES) is a method of storing and utilizing heat energy for various applications. It involves capturing thermal energy when it is abundant and releasing it when needed, enabling efficient heating and cooling processes and reducing energy consumption.
There are different types of thermal energy storage methods, but one common approach involves the use of phase change materials (PCMs). PCs can absorb or release large amounts of heat energy as they change their physical state, such as from solid to liquid or vice versa.
During the charging phase, heat is added to the PCM to melt it, storing the energy in the form of latent heat. When the stored heat is required, the PCM undergoes a phase change, releasing the stored heat, which can be used for space heating, water heating, or other industrial processes.
TES offers several advantages in terms of energy efficiency and cost savings. By storing excess thermal energy during off-peak hours or when renewable energy generation is high, TES systems can reduce the need for traditional heating or cooling devices during peak demand periods. This results in reduced energy consumption and lower energy costs.
TES can also enhance the efficiency of energy systems. For example, in solar thermal power plants, TES can store excess heat from the sun, allowing power generation to continue even when the sun is not shining. This results in a more consistent and reliable power output from renewable energy sources.
Additionally, TES provides an opportunity to shift energy demand from high-cost peak periods to low-cost off-peak periods. This can help to optimize energy usage, reduce strain on the grid, and promote a more balanced energy distribution throughout the day.
However, there are certain challenges and considerations associated with the implementation of TES. One challenge is the selection of appropriate PCMs based on the desired temperature range and the specific application requirements. PCMs need to be carefully chosen to ensure optimal performance and reliability.
Furthermore, TES systems may require the use of additional infrastructure, such as insulated tanks or heat exchangers, to store and transfer the thermal energy effectively. The design and integration of these components need to be carefully planned to maximize efficiency and minimize heat losses.
Research and development efforts are focused on improving the thermal energy storage systems by exploring new PCM materials, enhancing heat transfer mechanisms, and optimizing system designs. These advancements aim to make TES more cost-effective, reliable, and applicable in a wider range of applications.
Thermal energy storage is a valuable technology that enables efficient utilization of heat energy, reduces energy consumption, and promotes the integration of renewable energy sources. With ongoing advancements and innovation, TES holds promise for creating more sustainable and energy-efficient heating, cooling, and power generation systems.
Advancements in Energy Storage Technologies
The field of energy storage has witnessed significant advancements in recent years, driven by the increasing demand for clean and reliable energy solutions. These advancements have led to the development of more efficient, sustainable, and cost-effective energy storage technologies. Let’s explore some of the notable advancements:
- Lithium-ion Batteries: Lithium-ion batteries have undergone remarkable improvements in energy density, cycle life, and safety. This has been fueled by advancements in electrode materials, such as lithium iron phosphate and nickel-cobalt-aluminum oxide, and innovations in cell design and manufacturing processes. These advancements have led to the widespread adoption of lithium-ion batteries in portable electronics, electric vehicles, and grid-scale energy storage.
- Flow Batteries: Flow batteries have gained attention as a promising solution for long-duration energy storage. Advancements in flow battery technologies, such as vanadium redox flow batteries, zinc-bromine flow batteries, and organic flow batteries, have led to improved energy efficiency, longer cycle life, and greater scalability. These developments have positioned flow batteries as suitable options for grid-scale energy storage applications.
- Solid-State Batteries: Solid-state batteries are a next-generation battery technology that offers higher energy densities, faster charging capabilities, and improved safety compared to conventional lithium-ion batteries. Recent advancements in materials science, including the use of solid electrolytes and advanced electrode materials, have shown promise in overcoming the challenges associated with solid-state battery technology. These advancements pave the way for safer and more efficient energy storage solutions.
- Hydrogen Fuel Cells: Hydrogen fuel cells have made significant progress in recent years, particularly in transportation and stationary power applications. Advancements in fuel cell technology, such as improved catalyst materials, increased efficiency, and reduced costs, have made hydrogen fuel cells a viable option for zero-emission power generation and transportation. Hydrogen fuel cells offer the advantage of longer-range and quicker refueling times compared to traditional battery-powered vehicles.
- Advanced Thermal Energy Storage: Advancements in thermal energy storage (TES) include the development of high-performance phase change materials (PCMs) and the integration of TES systems with renewable energy technologies. These advancements have increased the efficiency and reliability of TES, allowing for better management of thermal energy in heating, cooling, and power generation applications.
- Emerging Technologies: Various emerging energy storage technologies are currently under development or in early stages of commercialization. These include sodium-ion batteries, aluminum-ion batteries, supercapacitors, and advanced flywheel systems. These technologies offer the potential for higher energy densities, faster charging rates, longer lifespans, and lower costs, paving the way for further advancements in energy storage.
Overall, advancements in energy storage technologies are driven by a combination of scientific research, innovative engineering, and market demand for more sustainable and efficient solutions. These advancements play a vital role in enabling the integration of renewable energy sources, improving grid stability, reducing carbon emissions, and providing reliable and resilient energy storage options for various applications.
Challenges and Limitations of Energy Storage
While energy storage holds immense potential for revolutionizing our energy infrastructure, there are several challenges and limitations that need to be addressed. These challenges include:
- Cost: One of the primary challenges of energy storage is the cost associated with implementing and operating storage systems. Despite significant cost reductions in recent years, many storage technologies still require high upfront investments, making it challenging to achieve widespread adoption. Continued research and development efforts are focused on improving the cost-effectiveness of energy storage technologies.
- Technology Maturity: Although there have been notable advancements in energy storage technologies, some are still relatively new and not yet fully matured. Emerging storage technologies, such as solid-state batteries and advanced flow batteries, face challenges related to scalability, cycle life, and commercial viability. Further research and development are necessary to overcome these limitations and facilitate their widespread deployment.
- Environmental Impact: The production, use, and disposal of energy storage technologies can have environmental implications. For example, some battery technologies require the extraction of raw materials with environmental and social consequences. Additionally, the improper disposal of batteries can lead to pollution and waste management challenges. It is crucial to minimize the environmental impact of energy storage technologies and improve their sustainability throughout their lifecycle.
- Storage Capacity and Duration: Energy storage systems have different storage capacities and durations, and not all technologies are suitable for long-term or large-scale energy storage needs. The development of high-capacity and long-duration energy storage solutions is crucial to support the integration of intermittent renewable energy sources and ensure grid stability during extended periods of high demand or low generation.
- Efficiency and Performance: Energy storage systems have varying efficiencies, with some loss of energy during the storage and release processes. Improving the round-trip efficiency of storage technologies is critical to maximize the amount of stored energy that can be recovered. Additionally, performance factors such as charge and discharge rates, power density, and lifespan need to be optimized for different applications.
- Infrastructure Limitations: The deployment of certain energy storage technologies may require the development or modification of infrastructure, such as energy transmission and distribution systems or specialized storage facilities. These infrastructure requirements can pose logistical challenges and may require significant investments and planning.
Addressing these challenges and limitations requires a multi-faceted approach involving research and development, policy support, market incentives, and stakeholder collaboration. Continued innovation, cost reduction, standardization, and supportive regulatory frameworks are necessary to overcome these hurdles and unlock the full potential of energy storage for a sustainable and resilient energy future.
Future Prospects and Applications of Energy Storage
The future of energy storage holds immense potential and is set to play a crucial role in our transition to a sustainable and decentralized energy system. Advancements in technology and increasing demand for clean energy solutions are driving the development of new applications and expanding the role of energy storage. Here are some future prospects for energy storage:
- Renewable Energy Integration: As the share of renewable energy sources in our energy mix continues to grow, energy storage will become increasingly important for managing the intermittent nature of these sources. By storing excess renewable energy during periods of high generation and releasing it during peak demand or low generation periods, energy storage enables a more reliable and stable integration of renewables into the grid.
- Microgrids and Off-Grid Systems: Energy storage will play a vital role in the development of microgrids and off-grid systems. These localized energy systems can combine renewable energy generation, energy storage, and smart grid technologies to provide reliable, resilient, and sustainable power supply to communities, remote areas, and critical infrastructure.
- Electric Vehicle (EV) Charging Infrastructure: With the rapid growth of electric vehicles, energy storage will be essential for EV charging infrastructure. By using energy storage solutions, such as fast-charging stations equipped with large-scale batteries or vehicle-to-grid (V2G) systems, the charging of electric vehicles can be managed more efficiently, reducing strain on the grid and enabling bidirectional energy flow between vehicles and the grid.
- Grid Stability and Resilience: Energy storage systems will continue to contribute to grid stability and resilience by providing frequency regulation, voltage support, and backup power during grid outages. By quickly responding to fluctuations in power supply and demand, energy storage enhances grid reliability and reduces the risk of blackouts or disruptions.
- Time-of-Use Energy Management: As energy markets embrace time-of-use pricing structures, energy storage can help consumers optimize their energy usage and reduce costs. Through energy storage systems, individuals and businesses can store electricity during times of lower prices and use it during peak hours when electricity costs are higher, resulting in significant savings and more efficient energy management.
- Decentralized Energy Systems: Energy storage enables the decentralization of energy systems, empowering individuals and communities to generate, store, and utilize their own renewable energy. This decentralized approach, combined with peer-to-peer energy trading facilitated by blockchain technology, provides opportunities for increased energy autonomy, reduced reliance on centralized grids, and enhanced energy resilience.
These are just a few potential applications for energy storage in the future. With ongoing advancements in technology, improving cost-effectiveness, and supportive policies, energy storage will continue to find innovative applications in various sectors, including industry, transportation, buildings, and agriculture.
Moreover, emerging technologies, such as advanced battery chemistries, hydrogen storage, and next-generation materials, hold promise for further enhancing the performance, efficiency, and sustainability of energy storage systems.
As we strive towards a carbon-neutral and sustainable future, energy storage will be a key enabler, offering flexibility, reliability, and increased utilization of renewable energy sources. It will revolutionize the way we generate, store, and consume energy, paving the way for a more resilient, decentralized, and efficient energy system.
Conclusion
Energy storage is a critical component of our modern energy infrastructure, offering numerous benefits and playing a vital role in our transition towards a sustainable and resilient energy future. The advancements in energy storage technologies have revolutionized the way we generate, store, and utilize energy, enabling the integration of renewable energy sources, improving grid stability, and providing reliable power supply.
Batteries, such as lithium-ion and flow batteries, have seen significant improvements in energy density, cycle life, and safety, making them essential for portable electronics, electric vehicles, and grid-scale energy storage. Pumped hydro storage utilizes the stored potential energy of water to generate electricity, while compressed air energy storage offers long-duration storage capabilities. Flywheels provide rapid response times and efficient power delivery, and thermal energy storage optimizes heating, cooling, and power generation processes.
The future prospects of energy storage are promising. Energy storage will be instrumental in the integration of renewable energy sources, such as solar and wind power, into the grid, ensuring a reliable and stable energy supply. Microgrids and off-grid systems will benefit from localized energy storage solutions, while electric vehicle charging infrastructure will rely on energy storage for efficient charging and bidirectional energy flows.
Energy storage also contributes to grid stability and resilience, providing backup power and frequency regulation to mitigate the impacts of power outages. Time-of-use energy management and decentralized energy systems empower consumers to optimize their energy usage, reduce costs, and enhance energy autonomy.
However, energy storage does face challenges and limitations, including the cost of implementation, technology maturity, environmental impact, storage capacity and duration, efficiency, and infrastructure requirements. Overcoming these challenges will require ongoing research and development, supportive policies, and collaborative efforts among stakeholders in the energy industry.
In conclusion, energy storage is a game-changer in the pursuit of a sustainable and decentralized energy system. As advancements continue and technology evolves, energy storage will become even more efficient, cost-effective, and versatile, enabling us to harness the full potential of renewable energy, enhance grid stability, and ensure a reliable and resilient energy supply for generations to come.
Frequently Asked Questions about How To Store Energy
Was this page helpful?
At Storables.com, we guarantee accurate and reliable information. Our content, validated by Expert Board Contributors, is crafted following stringent Editorial Policies. We're committed to providing you with well-researched, expert-backed insights for all your informational needs.
0 thoughts on “How To Store Energy”