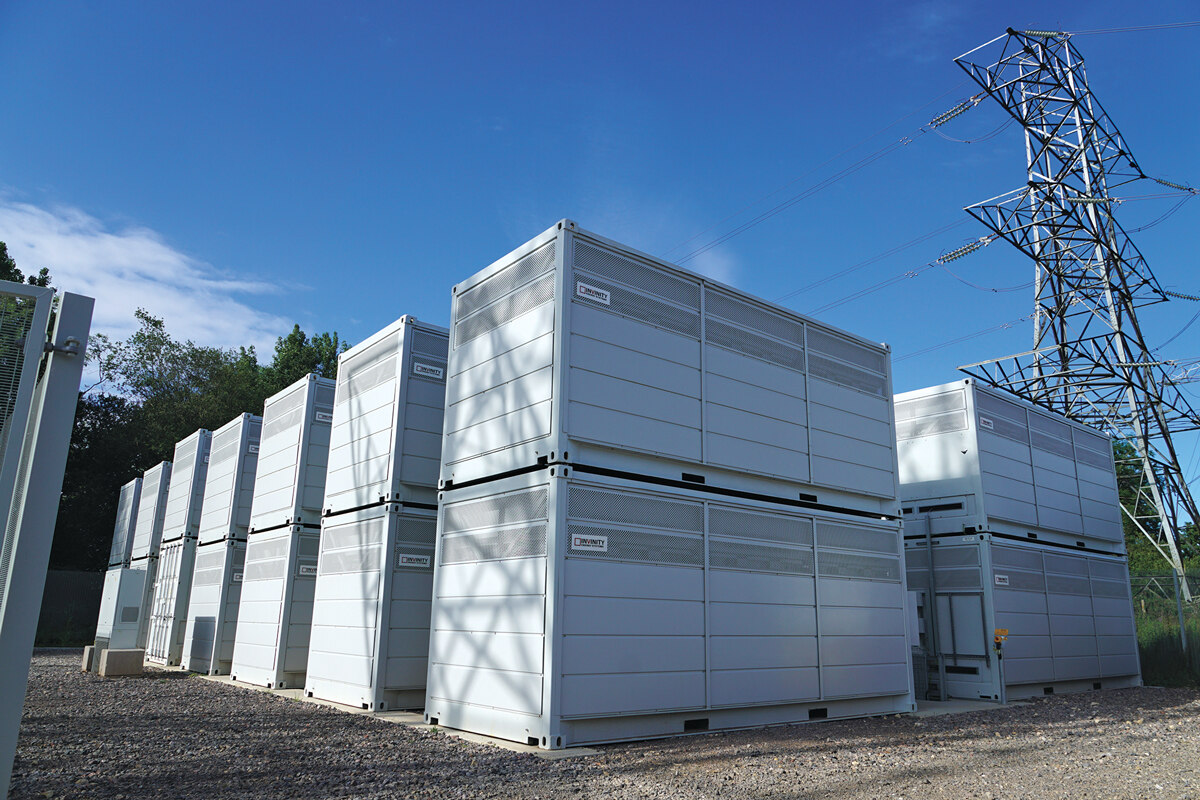
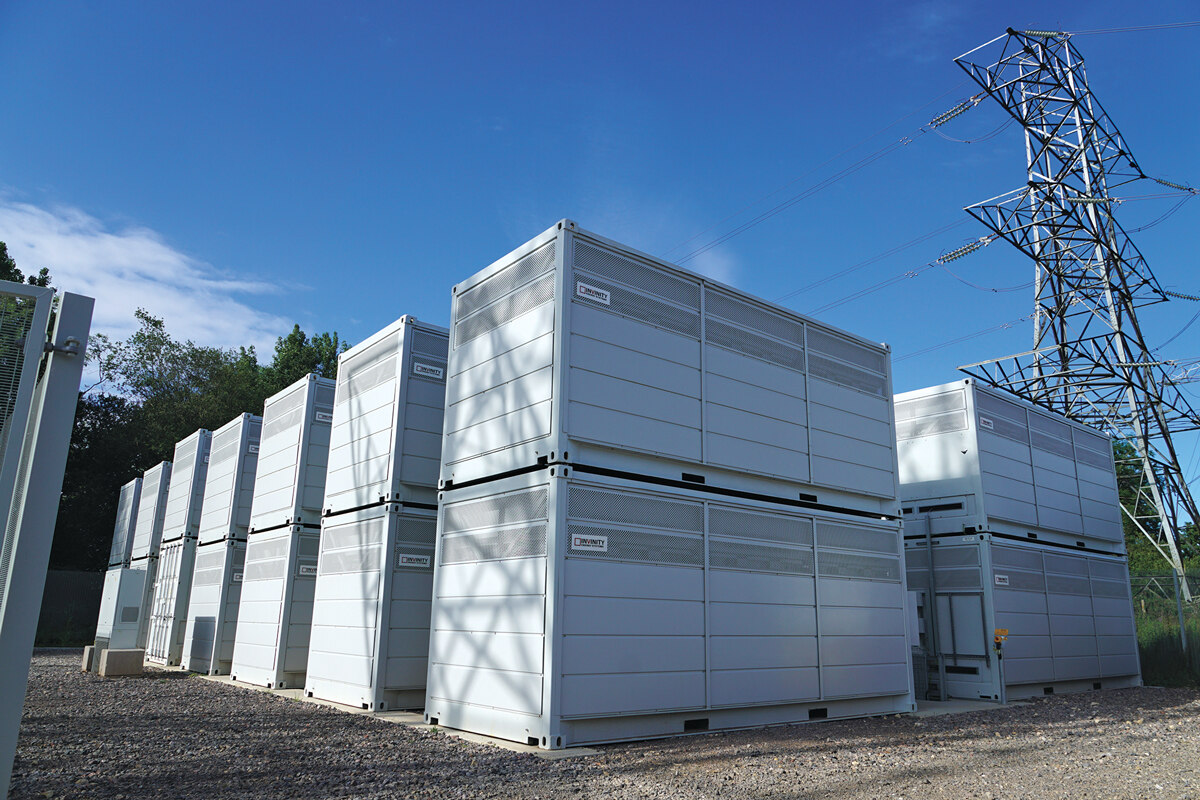
Articles
How To Store Energy In Battery
Modified: December 7, 2023
Learn how to efficiently store energy in batteries with our informative articles. Discover tips and techniques to maximize battery life and enhance energy storage capabilities.
(Many of the links in this article redirect to a specific reviewed product. Your purchase of these products through affiliate links helps to generate commission for Storables.com, at no extra cost. Learn more)
Introduction
Battery energy storage is a rapidly evolving technology that plays a crucial role in our modern society. As the demand for clean and reliable energy continues to rise, finding efficient and sustainable ways to store energy has become a top priority. Battery energy storage systems provide a solution by storing surplus energy during times of low demand and releasing it when needed, thereby balancing the supply and demand of electricity.
In this article, we will explore the various methods of storing energy in batteries, highlighting the advantages and disadvantages of different battery types and discussing the factors to consider when implementing battery energy storage systems. We will also delve into the charging and discharging techniques, safety considerations, and the diverse applications of battery energy storage.
With the growing emphasis on renewable energy sources such as wind and solar, battery energy storage has become a vital component in maintaining grid stability. In addition to providing a reliable backup power source, it enables the integration of intermittent renewable sources into the grid, allowing for a smoother and more efficient energy supply.
As the market for battery energy storage continues to expand, advancements in technology are driving improvements in energy density, lifespan, and cost-effectiveness. This article aims to provide a comprehensive overview of battery energy storage systems, empowering readers with a deeper understanding of the critical role they play in our energy transition.
So, whether you are a renewable energy enthusiast, a technician seeking to expand your knowledge, or simply curious about the future of energy storage, keep reading to discover the fascinating world of battery energy storage and its limitless potential.
Key Takeaways:
- Battery energy storage systems are revolutionizing the energy landscape, enabling renewable energy integration, grid stabilization, and electric mobility. Advancements in technology and safety measures are driving the transition to a cleaner and more sustainable energy future.
- From grid stability to electric vehicles, battery energy storage plays a vital role in diverse applications. Safety, proper maintenance, and adherence to best practices are essential for maximizing performance and mitigating risks in energy storage systems.
Read more: How To Store Batteries
Background on Battery Energy Storage
Battery energy storage has a long history, dating back to the late 18th century when Italian scientist Alessandro Volta invented the first true battery, known as the “voltaic pile.” This early battery consisted of alternating layers of zinc and copper discs separated by cardboard soaked in saltwater solution. While primitive by today’s standards, the voltaic pile laid the foundation for the development of modern batteries.
Over the years, battery technology has evolved significantly, driven by the increasing demand for portable power sources and the need for efficient energy storage systems. Today, batteries come in various forms and sizes, ranging from small rechargeable batteries used in smartphones to large-scale battery energy storage systems deployed in power grids.
Battery energy storage systems have gained immense popularity in recent years due to their versatility and ability to store and deliver electricity on-demand. These systems rely on chemical reactions to convert electrical energy into chemical energy and then convert it back to electrical energy when needed.
One of the primary drivers behind the surge in battery energy storage installations is the rapid integration of renewable energy sources into the grid. Wind and solar power, for example, are by nature intermittent, generating electricity when the sun shines or the wind blows. By storing surplus energy during periods of high supply and releasing it during times of high demand or low generation, battery energy storage helps balance the variability and unpredictability of renewable energy sources, improving grid reliability and stability.
Furthermore, battery energy storage systems offer several advantages over other conventional energy storage methods. Unlike pumped hydro storage, which requires specific geographical conditions, batteries can be deployed in diverse locations, ranging from residential homes to utility-scale installations. They are also fast-responding, allowing for rapid deployment of stored energy when grid demands suddenly spike.
Additionally, the declining costs of battery technologies and supportive government policies and incentives have contributed to the widespread adoption of battery energy storage systems. As battery production scales up and new advancements continue to emerge, the cost of batteries is expected to further decrease, making energy storage solutions even more accessible and economically viable.
In the upcoming sections, we will explore the different methods of storing energy in batteries, the advantages and disadvantages of various battery types, and the key considerations when implementing battery energy storage systems.
Various Methods of Storing Energy in Batteries
There are several different methods of storing energy in batteries, each with its own unique characteristics and suitability for specific applications. The choice of battery technology depends on factors such as energy density requirements, lifespan, safety considerations, and cost-effectiveness. Let’s explore some of the commonly used methods:
- Lithium-ion Batteries: Lithium-ion batteries are one of the most popular and widely used battery technologies. They offer high energy density, long cycle life, and low self-discharge rates. Lithium-ion batteries are commonly found in portable electronics, electric vehicles, and grid-scale energy storage systems. They provide a reliable and efficient method of storing and delivering energy.
- Lead-acid Batteries: Lead-acid batteries have been in use since the 19th century and are well-known for their reliability and affordability. These batteries are commonly used in applications such as automotive starting batteries and backup power systems. While lead-acid batteries have lower energy density compared to lithium-ion batteries, they are still widely used due to their robustness and low cost.
- Nickel-metal Hydride (NiMH) Batteries: NiMH batteries were once popular in consumer electronics, but their usage has declined with the rise of lithium-ion batteries. However, they are still used in certain applications such as hybrid electric vehicles (HEVs) and power tools. NiMH batteries offer a relatively high energy density and are environmentally friendly compared to other battery chemistries.
- Flow Batteries: Flow batteries are a unique type of battery where energy is stored in chemical solutions outside the battery cells. These solutions, known as electrolytes, flow through electrodes, producing electricity through chemical reactions. Flow batteries offer the advantage of being able to independently scale energy and power capacities and have long cycle life. They are commonly used in large-scale energy storage applications.
- Solid-state Batteries: Solid-state batteries are an emerging technology that holds great promise in the field of energy storage. Instead of using a liquid or gel electrolyte, solid-state batteries utilize solid electrolytes, improving safety and energy density. They have the potential to revolutionize battery technology by providing higher energy density, faster charging times, and enhanced safety.
Each method of storing energy in batteries has its own set of advantages and disadvantages, making them suitable for different applications. Factors such as energy requirements, discharge rates, cycling needs, and cost considerations need to be evaluated when selecting the appropriate battery technology for a specific use case.
In the next section, we will explore the advantages and disadvantages of different battery types to gain a better understanding of their capabilities and limitations in energy storage applications.
Lithium-ion Batteries
Lithium-ion batteries are the gold standard in battery technology, known for their high energy density, long cycle life, and excellent performance. These batteries are widely used in a variety of applications, including portable electronics, electric vehicles, and grid-scale energy storage systems.
One of the primary advantages of lithium-ion batteries is their impressive energy density. These batteries can store a significant amount of electrical energy in a compact and lightweight design, making them ideal for applications where space is limited, such as smartphones or electric vehicles. The high energy density of lithium-ion batteries also allows for longer-lasting battery life, as more energy can be stored without sacrificing performance.
Another key advantage of lithium-ion batteries is their long cycle life. Unlike some other battery chemistries, lithium-ion batteries can endure a large number of charge and discharge cycles without significant degradation in capacity or performance. This makes them highly durable and cost-effective over the long term, as they can be used for many years without needing frequent replacements.
In addition, lithium-ion batteries offer a high voltage output and excellent discharge characteristics. They can deliver energy at a consistent rate, ensuring a steady power supply to the connected devices or systems. This feature is crucial in applications where a stable and reliable source of electricity is essential, such as residential energy storage or grid stabilization.
Lithium-ion batteries also have a low self-discharge rate, meaning they retain their stored energy for extended periods when not in use. This allows for longer standby times and reduces the need for frequent recharging, making them convenient for devices that may not be used frequently but need to be ready for use at any time.
However, it is important to note that lithium-ion batteries do have some drawbacks. Safety is one such concern, as these batteries can be prone to thermal runaway, which can lead to overheating and, in extreme cases, even fires or explosions. Manufacturers mitigate these risks through careful design and incorporation of safety mechanisms, but proper handling and monitoring of lithium-ion batteries are essential to prevent incidents.
Another consideration is the cost of lithium-ion batteries, which can be relatively high compared to other battery chemistries. However, as the demand for these batteries continues to increase, advancements in technology and economies of scale are driving down costs, making lithium-ion batteries more affordable over time.
Overall, lithium-ion batteries have revolutionized the world of energy storage, providing high energy density, long cycle life, and reliable performance. As technology continues to improve and costs decrease, lithium-ion batteries will continue to play a vital role in powering our portable devices and driving the clean energy transition.
Lead-acid Batteries
Lead-acid batteries have a long history and are one of the oldest and most established battery technologies. They are widely used in various applications, including automotive starting batteries, backup power systems, and off-grid renewable energy systems.
One of the key advantages of lead-acid batteries is their affordability. Compared to other battery chemistries, such as lithium-ion, lead-acid batteries have a lower upfront cost, making them a cost-effective option for many applications. This makes lead-acid batteries particularly attractive for uses where a large amount of energy storage is required but cost constraints are in place.
Lead-acid batteries are also known for their robustness and durability. They can withstand deep discharges and high discharge rates without significant loss in performance. This makes them suitable for applications where occasional high-power demands are necessary, such as starting an engine or providing backup power during an outage.
Furthermore, lead-acid batteries have a long-standing track record of reliable performance, proven through their widespread use in automotive applications. They have been extensively tested and improved over the years, ensuring their reliability and efficiency.
Despite their advantages, lead-acid batteries do have some limitations. One of the major drawbacks is their relatively low energy density compared to other battery types. This means lead-acid batteries are bulkier and heavier for a given energy storage capacity. As a result, they are less suitable for portable and space-constrained applications.
Lead-acid batteries also have a shorter cycle life compared to lithium-ion batteries. They are usually designed for shallow cycling and may experience reduced capacity over time if subjected to deep discharges. Regular maintenance, like maintaining proper electrolyte levels and monitoring charging voltages, is required to maximize their lifespan and performance.
Additionally, lead-acid batteries contain toxic substances such as sulfuric acid and lead, which raises environmental concerns. Proper disposal and recycling practices must be followed to prevent pollution and ensure the safe disposal of these batteries.
Despite the emergence of newer battery technologies, lead-acid batteries remain a reliable and cost-effective option for many applications, particularly those that require robustness, large energy storage capacities, and affordability. Ongoing research and development efforts aim to improve their energy density, cycle life, and environmental impact, ensuring they continue to serve as a viable energy storage solution for years to come.
Read more: How To Store A Battery
Nickel-metal Hydride (NiMH) Batteries
Nickel-metal Hydride (NiMH) batteries have been widely used in various applications, although their popularity has diminished with the emergence of lithium-ion batteries. These batteries offer several advantages such as high energy density, enhanced safety, and environmental friendliness.
One notable advantage of NiMH batteries is their relatively high energy density. Compared to older battery chemistries like lead-acid batteries, NiMH batteries offer a higher capacity to store energy, allowing for longer-lasting power in portable devices such as laptops, digital cameras, and handheld tools.
In terms of safety, NiMH batteries are considered safer than some other battery types, like lithium-ion batteries. They have a lower risk of thermal runaway, making them less prone to overheating or catching fire. This safety aspect is particularly crucial for applications where battery reliability is of utmost importance, such as medical devices or safety systems.
Another significant advantage of NiMH batteries is their environmental friendliness. They are less harmful to the environment compared to older battery chemistries since they do not contain toxic materials like cadmium, which is found in older nickel-cadmium (NiCd) batteries. This characteristic has led to a gradual shift from NiCd to NiMH batteries in many applications.
However, it’s important to note that NiMH batteries also have their limitations. One of the main drawbacks is their lower energy density compared to lithium-ion batteries. This means that for a given physical size, NiMH batteries can store less energy, making them less suitable for applications that require long-lasting, high-energy performance.
NiMH batteries also suffer from self-discharge, resulting in a loss of stored capacity over time, even when not in use. This can be a significant drawback in applications where long-term storage without regular recharging is essential. However, advancements in technology have helped reduce their self-discharge rates compared to older NiCd batteries.
Furthermore, NiMH batteries have a lower cycle life compared to lithium-ion batteries, meaning they can endure fewer charge and discharge cycles before experiencing reduced capacity. Regular maintenance and proper charging techniques are necessary to maximize their lifespan and maintain their performance.
In recent years, the decline in NiMH battery usage can be attributed to the emergence of lithium-ion batteries, which offer higher energy density, longer cycle life, and faster charging capabilities. However, NiMH batteries still have their place in certain applications where cost-effectiveness, safety, and environmental sustainability are crucial factors.
The ongoing research and development efforts aim to further enhance the energy density and cycle life of NiMH batteries, making them a more attractive option for various energy storage needs.
Flow Batteries
Flow batteries are a unique type of battery technology that offers several advantages over traditional batteries. In flow batteries, the energy is stored externally in chemical solutions known as electrolytes, which flow through the battery during operation.
One of the key advantages of flow batteries is their ability to independently scale the energy and power capacities. Unlike other battery technologies where energy and power are directly proportional, flow batteries allow for the decoupling of these two characteristics. This flexibility makes flow batteries highly versatile and suitable for a wide range of applications that require varying energy and power requirements.
Flow batteries also offer a significantly longer cycle life compared to many other battery chemistries. Since the active materials are stored externally in the electrolytes, flow batteries do not experience degradation in the same way that traditional batteries do. This allows for a longer lifespan and ensures that flow batteries can endure a large number of charge and discharge cycles without significant losses in capacity.
Moreover, flow batteries excel in their high energy storage capacity. The amount of energy stored in a flow battery is determined by the size of the external electrolyte tanks, allowing for the storage of large amounts of energy over an extended period. This makes flow batteries particularly suitable for long-duration energy storage applications, such as grid-scale energy storage or renewable energy integration.
Another advantage of flow batteries is their inherent safety. Since the active materials are stored externally and only flow through the battery during operation, the risk of thermal runaway or catastrophic failure is significantly reduced. This enhances the overall safety of flow battery systems, making them a reliable choice for applications where safety is a top priority.
However, flow batteries do have some limitations. One of the main drawbacks is their relatively lower energy density compared to some other battery technologies. The large external storage of electrolyte solutions requires more physical space, making flow batteries less suitable for applications where size and weight constraints are critical, such as portable electronic devices.
Flow batteries also face challenges in terms of efficiency and cost. The process of pumping electrolytes through the battery can introduce energy losses, resulting in lower round-trip efficiency compared to traditional batteries. Additionally, the components required for flow battery systems, such as tanks, pumps, and membranes, can contribute to higher upfront costs. However, ongoing research and development efforts aim to improve the efficiency and cost-effectiveness of flow battery technology.
Despite these limitations, flow batteries are gaining increasing attention and market adoption, particularly in large-scale energy storage applications. Their unique scalability, long cycle life, safety features, and high energy storage capacity make them a promising solution for the future of sustainable energy storage.
To store energy in a battery, make sure to keep it at a moderate temperature (around 20-25°C) and avoid fully discharging or overcharging it to prolong its lifespan.
Solid-state Batteries
Solid-state batteries are an emerging technology that holds great promise for the future of energy storage. Unlike traditional batteries that use liquid or gel electrolytes, solid-state batteries utilize solid electrolytes, offering several advantages over their conventional counterparts.
One of the primary advantages of solid-state batteries is their higher energy density. By using a solid-state electrolyte, these batteries can store more energy in a smaller package, making them ideal for applications where size and weight are critical factors. The higher energy density of solid-state batteries also translates to longer-lasting power and increased runtimes in portable devices.
Another key advantage of solid-state batteries is their improved safety. The absence of flammable liquid electrolytes significantly reduces the risk of thermal runaway and related safety concerns. This makes solid-state batteries inherently safer, especially in applications that require high levels of reliability and where accidents or failures could have serious consequences.
Solid-state batteries also offer faster charging capabilities compared to traditional batteries. The solid electrolyte allows for faster ion transport, enabling rapid charging without compromising battery lifespan or safety. This feature is particularly valuable in applications where quick charging is essential, such as electric vehicles or consumer electronics.
Furthermore, solid-state batteries exhibit greater stability and longer cycle life. The solid electrolyte minimizes electrode degradation, allowing for a greater number of charge and discharge cycles without significant loss in capacity or performance. This results in more durable batteries that can withstand prolonged use and offer extended lifespans.
Despite their many advantages, solid-state batteries still face some challenges. One of the main hurdles is the high production cost associated with the manufacturing of solid-state electrolytes. Research and development efforts are focused on finding cost-effective and scalable production methods to make solid-state batteries more commercially viable.
Additionally, the performance of current solid-state batteries can be limited by low ionic conductivity at the solid electrolyte-electrode interface. Scientists and engineers are actively working on improving the performance and efficiency of solid-state batteries by enhancing the conductivity and stability of the solid electrolyte materials.
Despite these challenges, solid-state batteries are considered the next frontier in energy storage technology. Their potential for higher energy density, improved safety, faster charging, and enhanced stability makes them a highly promising solution for a wide range of applications, including electric vehicles, grid-scale storage, and portable electronics. Continued research and development efforts are expected to unlock the full potential of solid-state batteries, paving the way for a greener and more efficient energy future.
Advantages and Disadvantages of Different Battery Types
When it comes to energy storage, different battery technologies offer a range of advantages and disadvantages. Understanding the strengths and weaknesses of each battery type is crucial in selecting the most suitable option for specific applications. Let’s examine the key advantages and disadvantages of different battery types:
- Lithium-ion Batteries:
- Advantages:
- High energy density, allowing for more compact designs and longer battery life.
- Long cycle life, providing a durable and cost-effective energy storage solution.
- Quick charging capabilities, enabling rapid charging of devices.
- Low self-discharge rate, making them convenient for devices that are infrequently used.
- Disadvantages:
- Potential safety concerns if mishandled or subjected to extreme conditions.
- Higher upfront cost compared to some other battery technologies.
- Environmental concerns regarding extraction and disposal of lithium.
- Lead-acid Batteries:
- Advantages:
- Reliable and affordable option, suitable for various applications.
- Robustness and durability, with the ability to handle deep discharges and high discharge rates.
- Well-established and widely used technology.
- Disadvantages:
- Lower energy density compared to some other battery types.
- Shorter cycle life compared to lithium-ion batteries.
- Contains toxic substances, requiring proper disposal and recycling methods.
- Nickel-metal Hydride (NiMH) Batteries:
- Advantages:
- High energy density, offering longer-lasting power in portable devices.
- Enhanced safety compared to some other battery chemistries.
- Environmentally friendly, without the use of toxic materials like cadmium found in older battery types.
- Disadvantages:
- Lower energy density compared to lithium-ion batteries.
- Self-discharge over time, requiring regular recharging.
- Shorter cycle life compared to some newer battery technologies.
- Flow Batteries:
- Advantages:
- Independent scaling of energy and power capacities, offering flexibility in various applications.
- Long cycle life, making them durable and cost-effective.
- High energy storage capacity, suitable for long-duration energy storage needs.
- Disadvantages:
- Lower energy density compared to some conventional battery technologies.
- Higher upfront costs due to additional components like tanks and pumps.
- Potential efficiency losses in the flow process.
- Solid-state Batteries:
- Advantages:
- Higher energy density, allowing for more compact designs and longer-lasting power.
- Improved safety due to the absence of flammable liquid electrolytes.
- Faster charging capabilities and longer cycle life.
- Disadvantages:
- Higher production costs due to the complex manufacturing processes.
- Challenges in achieving high ionic conductivity at the solid electrolyte-electrode interface.
It’s important to consider these advantages and disadvantages carefully when selecting the appropriate battery technology for specific applications. Each battery type has its own trade-offs, and it’s crucial to assess their suitability based on factors such as energy requirements, size constraints, safety considerations, lifespan, and cost-effectiveness.
By understanding the unique characteristics of different battery types, we can make informed decisions that align with our energy storage needs while considering factors such as performance, cost, and sustainability.
Read also: 15 Best Battery Storage For 2024
Factors to Consider when Storing Energy in Batteries
When it comes to storing energy in batteries, several important factors should be considered. These factors help determine the suitability of a battery technology for a specific application and ensure efficient and effective energy storage. Let’s explore some key considerations:
- Energy Density: Energy density is a crucial factor when selecting a battery for energy storage. It refers to the amount of energy a battery can store relative to its size and weight. Higher energy density batteries are desirable for applications where space and weight constraints are critical, allowing for more energy storage in a compact form.
- Power Rating: The power rating of a battery is another important factor to consider. It represents the ability of a battery to deliver energy at a specific rate. Applications that require high-power demands, such as electric vehicles or grid-scale energy storage systems, need batteries with a higher power rating to meet the instantaneous energy requirements.
- Cycle Life: Cycle life refers to the number of charge and discharge cycles a battery can endure before experiencing a significant loss in capacity. For applications that require frequent cycling, such as renewable energy integration or electric vehicles, batteries with a longer cycle life are preferred to ensure longevity and cost-effectiveness.
- Safety: Safety is a critical consideration when storing energy in batteries. Different battery chemistries have varying safety profiles, and the application’s requirements should align with the safety characteristics of the chosen battery technology. Measures should be implemented to mitigate risks associated with thermal runaway, overcharging, or mishandling of batteries.
- Cost: The cost of a battery system plays a significant role in determining its viability for an application. Factors such as battery chemistry, manufacturing processes, and scale of production affect the overall cost. It’s important to evaluate the cost-effectiveness of a battery technology in relation to its performance, durability, and estimated lifespan.
- Environmental Impact: The environmental impact of battery technologies should also be considered. This includes the extraction and production of raw materials, as well as the disposal and recycling of batteries. Batteries with lower environmental footprints, such as those with reduced heavy metal content or more recyclable components, are preferable for sustainable energy storage solutions.
- Performance in Different Conditions: The performance of batteries can vary under different operating conditions, such as temperature extremes or high humidity. It’s important to consider how battery technologies perform in specific environments and how they are affected by such conditions. Some batteries may have temperature limitations or require additional thermal management systems to optimize their performance.
- Scalability: Scalability is an essential factor, especially for applications that require large-scale energy storage solutions. The ability to scale the energy and power capacities of the battery system to meet changing demands is crucial. For example, grid-scale energy storage systems may require the integration of multiple battery units to achieve the desired capacity.
- Maintenance and Lifespan: The maintenance requirements and expected lifespan of a battery technology should be assessed. Some batteries may require regular maintenance, such as electrolyte level monitoring or cell balancing, to ensure optimal performance and prolong their lifespan. Understanding the maintenance needs and expected lifespan helps in planning for the operational and replacement costs of the battery system.
By carefully considering these factors, one can select the most appropriate battery technology for energy storage needs. The specific requirements of the application, including energy density, power rating, cycle life, safety, cost, environmental impact, performance in different conditions, scalability, and maintenance needs, play critical roles in determining the optimal battery solution. Taking these factors into account ensures an energy storage system that meets performance requirements, is cost-effective, and aligns with sustainability goals.
Charging and Discharging Techniques
Charging and discharging techniques are crucial aspects of energy storage systems, determining how efficiently and effectively energy is stored and retrieved from batteries. These techniques play a vital role in maximizing battery performance, ensuring longevity, and optimizing the overall energy storage system. Let’s explore some common charging and discharging techniques:
- Constant Current (CC): In constant current charging, a steady current flow is maintained during the initial charging phase. This technique allows the battery to rapidly charge up to a certain voltage threshold, ensuring a swift charging process. However, as the battery approaches its maximum capacity, the charging current is gradually reduced to avoid overcharging and potential damage to the battery.
- Constant Voltage (CV): Once the battery reaches the desired voltage threshold, constant voltage charging is employed. In this technique, the voltage across the battery terminals is maintained at a specific level while the charging current is gradually lowered. Constant voltage charging helps prevent overcharging, allowing the battery to reach its full capacity slowly and safely.
- Trickle Charging: Trickle charging is a low-rate charging technique used to maintain the charge level in batteries that are not frequently used. A small current is continuously supplied to the battery, compensating for the self-discharge and ensuring that it stays charged and ready for use. Trickle charging is commonly employed in applications like standby power systems or battery-operated devices with intermittent use.
- Pulse Charging: Pulse charging involves delivering short bursts of high-current pulses to the battery during the charging process. These pulses help break down any crystalline formations or sulfation that may have occurred on the battery electrodes, improving performance and extending battery life. Pulse charging is often used for lead-acid batteries or batteries that have been subjected to long periods of inactivity.
- Fast Charging: Fast charging techniques aim to recharge batteries in a significantly shorter time compared to standard charging methods. This typically involves higher charging currents and specialized charging algorithms that balance the speed of charging with considerations for battery health and safety. Fast charging is commonly used in applications where quick battery recharge is essential, such as electric vehicles or mobile devices.
- Depth of Discharge (DOD) Management: Depth of discharge management focuses on controlling the extent to which a battery is discharged during its usage. By limiting the depth of discharge, the battery’s cycle life can be extended. This technique helps prevent excessive wear and stress on the battery, ensuring optimal performance and longevity.
- Load-leveling: Load-leveling is a discharging technique employed in grid-scale energy storage systems. During times of high electricity demand, the stored energy from the batteries is discharged to the grid, helping alleviate strain on the power system. Load-leveling ensures a more stable and reliable energy supply by balancing the supply and demand, particularly during peak usage periods.
- Peak Shaving: Peak shaving is a technique primarily used in commercial and industrial settings to reduce electricity costs. Batteries store excess energy during times of low demand and discharge it during times of high demand, effectively reducing the peak power consumption from the grid. This helps avoid peak demand charges imposed by utility companies, resulting in significant cost savings.
The choice of charging and discharging techniques depends on various factors, including battery chemistry, specific application requirements, and preferences for performance, lifespan, and efficiency. Careful consideration of these techniques ensures that the battery is charged and discharged optimally, maximizing its capacity, efficiency, and overall lifespan.
Safety Considerations and Best Practices
Safety is of utmost importance when it comes to utilizing battery energy storage systems. Implementing proper safety measures and adhering to best practices ensures the prevention of accidents, minimizes risks, and maximizes the performance and lifespan of the batteries. Here are some key safety considerations and best practices to follow:
- Battery Management System (BMS): Implementing a reliable battery management system is crucial for monitoring and maintaining the optimal performance of the battery system. A BMS helps in controlling parameters such as temperature, voltage, and current, ensuring safe charging and discharging operations. It also provides protection against overcharging, over-discharging, and excessive temperatures, helping to prevent potential hazards.
- Proper Ventilation and Cooling: Batteries generate heat during charging and discharging processes. Adequate ventilation and cooling systems must be in place to dissipate excess heat and maintain the battery within an optimal temperature range. This helps prevent thermal runaway, which can lead to fire hazards and decreased battery performance. Regular inspection of cooling systems is essential to ensure their proper functionality.
- Protection Against Overcharging and Over-discharging: Overcharging and over-discharging are detrimental to battery health and safety. Proper charging algorithms, as well as voltage and current regulation, should be implemented to prevent these conditions. Constant monitoring of the battery voltage and state of charge is necessary to avoid exceeding safe limits, which can cause irreversible damage and compromise safety.
- Preventing Physical Damage: Protecting batteries from physical damage is crucial to maintain their integrity and prevent hazardous situations. Batteries should be securely mounted, protected from vibrations, and shielded from potential impacts. Proper handling procedures, such as avoiding dropping or mishandling batteries, should be followed to prevent damage to the battery cells or terminals.
- Fire Prevention and Suppression: Fire safety measures should be in place to mitigate the risk of accidents. This includes the installation of fire detection systems, fire suppression equipment, and the presence of suitable fire extinguishers in the vicinity of the battery installation. Adequate training should be provided to personnel on fire prevention and emergency response procedures.
- Regular Inspection and Maintenance: Regular inspection and maintenance are important to ensure the ongoing safety and performance of the battery system. This includes monitoring battery performance parameters, checking for signs of corrosion or leakage, and inspecting electrical connections, cables, and insulation. Timely maintenance and replacement of faulty components help prevent potential safety hazards.
- Compliance with Regulations and Codes: Adhering to safety regulations, local codes, and industry standards is essential for safe battery storage operations. Familiarize yourself with relevant safety guidelines and consult with professionals to ensure compliance with electrical, building, and fire codes. Regular audits and inspections can help identify and address any safety concerns or non-compliance issues.
- Training and Education: Proper training and education of personnel involved in the operation, maintenance, and handling of the battery system are critical. This includes training on battery safety protocols, emergency response procedures, and the proper use of personal protective equipment (PPE) when working with batteries. Regular refresher courses and awareness campaigns help reinforce safety practices and promote a safety-conscious culture.
By following these safety considerations and best practices, the risk of accidents, such as thermal runaway, fires, or other safety hazards, can be significantly minimized. Prioritizing safety ensures the well-being of personnel, preserves the integrity of the battery system, and promotes the efficient and effective use of battery energy storage.
Applications of Battery Energy Storage
Battery energy storage systems have a wide range of applications across various sectors, contributing to a more sustainable and reliable energy landscape. From enhancing grid stability to providing backup power and enabling the integration of renewable energy sources, batteries play a crucial role in shaping our energy future. Let’s explore some key applications of battery energy storage:
- Renewable Energy Integration: One of the primary applications of battery energy storage is the integration of renewable energy sources into the grid. Renewable sources like solar and wind are intermittent and often generate excess energy during off-peak hours. Battery storage systems capture this surplus energy and deliver it to the grid when demand is high or when renewable generation is insufficient, ensuring a smoother and more stable energy supply.
- Grid Stabilization and Frequency Regulation: Battery energy storage systems provide valuable services to maintain grid stability and regulate frequency. They can rapidly respond to fluctuations in electricity demand or supply, injecting or absorbing power as needed, thereby balancing the grid and ensuring reliable supply to consumers. This is particularly valuable in situations where traditional power plants may take time to adjust their output.
- Peak Load Shaving and Demand Management: Battery energy storage is employed in commercial and industrial settings to manage peak power demand. By storing excess energy during off-peak periods and discharging it during peak demand hours, batteries help reduce electricity costs and alleviate strain on the power grid. This application is beneficial for businesses looking to reduce their electricity bills while maintaining uninterrupted operations.
- Microgrids and Remote Power Systems: Battery energy storage plays a crucial role in the development of microgrids and remote power systems. These systems integrate renewable energy sources and storage to provide reliable, clean power to areas without access to a traditional grid. Battery storage ensures a continuous electricity supply, even during periods of low renewable generation or when the main grid experiences outages.
- Uninterruptible Power Supply (UPS): Battery energy storage is commonly used in UPS systems to provide backup power during outages. UPS systems ensure continuous operation and protect critical equipment, such as data centers, hospitals, and telecommunications infrastructure, from power disruptions. Batteries serve as a reliable and fast-responding backup power source, bridging the gap until primary power supply is restored.
- Electric Vehicles (EVs): Battery energy storage is the driving force behind the electrification of transportation. Lithium-ion batteries power electric vehicles, offering a clean and sustainable alternative to traditional combustion engines. The high energy density and fast-charging capabilities of batteries enable longer driving ranges and rapid recharging, making electric vehicles a viable and eco-friendly transportation option.
- Residential Energy Storage: Battery energy storage systems are increasingly being adopted in residential settings. Homeowners can store excess solar energy generated during the day and utilize it during the evening or during power outages. Residential energy storage systems provide greater energy independence, reduced reliance on the grid, and potential cost savings by optimizing self-consumption of renewable energy.
These applications illustrate the versatility and importance of battery energy storage in today’s energy landscape. From balancing the variability of renewables to providing backup power and enabling the transition to electric mobility, batteries are at the forefront of shaping a more sustainable, reliable, and resilient energy future.
Read more: How To Store Batteries At Home
Conclusion
Battery energy storage has emerged as a critical technology for our modern society, revolutionizing the way we store and utilize energy. With their high energy density, long cycle life, and versatile applications, batteries have become an integral part of the transition towards a cleaner and more sustainable energy landscape.
From grid stabilization and renewable energy integration to backup power and electric transportation, battery energy storage systems have proven their value across various sectors. They support the integration of intermittent renewable sources, enhance grid stability, reduce peak demand, and provide reliable power during outages.
Advancements in battery technologies, particularly in areas such as lithium-ion batteries, have driven improvements in energy density, power capabilities, and charging efficiency. Lower costs, coupled with supportive government policies and incentives, have encouraged widespread adoption, further boosting the market for battery energy storage systems.
However, while the potential of battery energy storage is vast, safety considerations, proper maintenance, and adherence to best practices are essential. Ensuring the safe operation of battery systems, implementing effective charging and discharging techniques, and following recommended safety protocols are crucial for maximizing battery performance and mitigating potential risks.
As we continue to embrace the clean energy transition, battery energy storage will play a crucial role in securing our energy future. Continued research and development efforts aim to improve battery efficiency and longevity, enhance safety features, and reduce costs, making energy storage solutions more accessible and sustainable for individuals, businesses, and utilities alike.
In conclusion, battery energy storage systems are driving the transformation of our energy systems, enabling increased renewable energy utilization, strengthening grid resilience, and revolutionizing transportation. The future holds great promise for battery technologies, and their ongoing advancements will pave the way for a greener, more efficient, and decentralized energy landscape.
Frequently Asked Questions about How To Store Energy In Battery
Was this page helpful?
At Storables.com, we guarantee accurate and reliable information. Our content, validated by Expert Board Contributors, is crafted following stringent Editorial Policies. We're committed to providing you with well-researched, expert-backed insights for all your informational needs.
0 thoughts on “How To Store Energy In Battery”