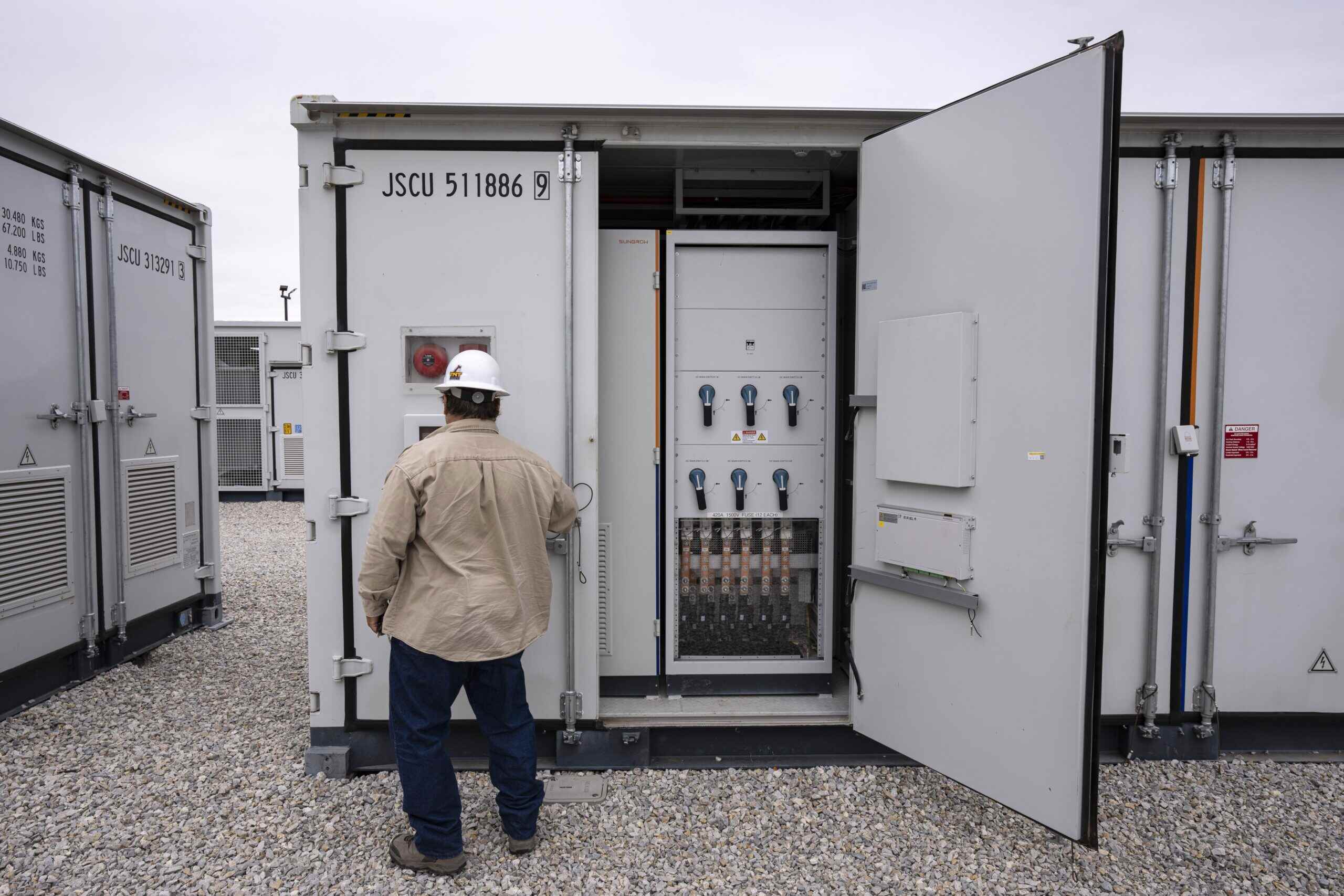
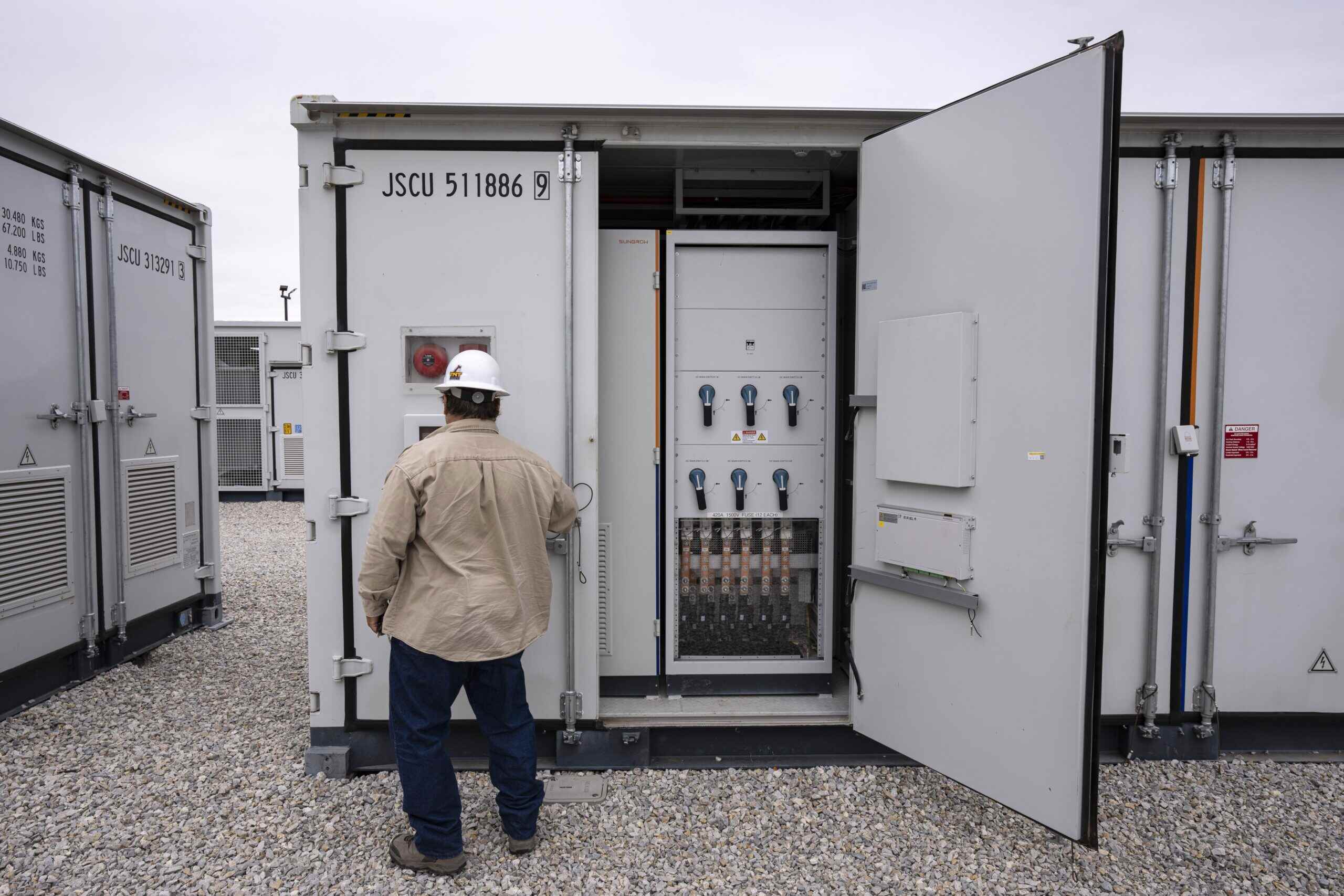
Articles
How To Store Electrical Energy
Modified: January 9, 2024
Discover the best articles on how to store electrical energy efficiently. Learn about various techniques and technologies used for effective energy storage.
(Many of the links in this article redirect to a specific reviewed product. Your purchase of these products through affiliate links helps to generate commission for Storables.com, at no extra cost. Learn more)
Introduction
Welcome to the world of electrical energy storage! In today’s rapidly advancing technological landscape, the demand for efficient and reliable energy storage solutions is on the rise. As renewable energy sources gain more prominence, the need to store the intermittent power they generate becomes increasingly critical. Electrical energy storage plays a vital role in balancing the supply and demand of electricity, ensuring grid stability, and enabling the transition to a more sustainable energy future.
Electrical energy storage refers to the process of capturing and storing electrical energy for later use. It allows us to save excess energy during times of low demand and release it when demand is high or when renewable sources are not readily available. By doing so, energy storage systems help to optimize the utilization of renewable resources, reduce greenhouse gas emissions, and enhance the overall resilience and reliability of power systems.
There are various types of electrical energy storage technologies available, each with its own unique characteristics and applications. From batteries and capacitors to flywheel energy storage and pumped hydro storage, the options are diverse and constantly evolving. In this article, we will explore some of the most commonly used energy storage technologies and their respective benefits and drawbacks.
Additionally, we will delve into advancements in the field of electrical energy storage, such as emerging technologies and innovative approaches that aim to overcome existing limitations and further enhance the efficiency and affordability of energy storage systems. We will also discuss key considerations for implementing and operating efficient energy storage solutions, including factors such as cost, scalability, environmental impact, and safety.
So, whether you are a renewable energy enthusiast, a power systems engineer, or simply curious about the future of electricity generation and storage, this article will provide you with valuable insights and knowledge about the fascinating world of electrical energy storage. Let’s dive in and explore the technologies and advancements that are shaping the way we store electrical energy!
Key Takeaways:
- Electrical energy storage is essential for balancing supply and demand, integrating renewable energy, and ensuring grid stability. From batteries to thermal storage, diverse technologies offer unique benefits for a sustainable energy future.
- Advancements in energy storage, such as lithium-ion batteries and hybrid systems, drive efficiency and sustainability. Considerations like system design, grid compatibility, and safety are crucial for maximizing the benefits of energy storage.
Read more: Which Is Better Gas Or Electric Water Heater
Importance of Electrical Energy Storage
Electrical energy storage plays a crucial role in modern society, as it addresses some of the most pressing challenges in the energy sector. Here are some key reasons highlighting the importance of electrical energy storage:
- Balancing Supply and Demand: One of the greatest challenges in the power industry is matching electricity supply with demand. Electrical energy storage allows us to capture excess energy during periods of low demand and release it during peak demand periods. This ensures a more stable and reliable supply of electricity, reducing the risk of blackouts and brownouts.
- Integration of Renewable Energy: Renewable energy sources such as solar and wind power are intermittent by nature, meaning their output fluctuates depending on weather conditions. By using electrical energy storage, we can capture and store excess energy generated by renewable sources for use when the sun isn’t shining or the wind isn’t blowing. This enhances the overall reliability of renewable energy systems and enables a smoother integration into the grid.
- Grid Stability and Resilience: Energy storage systems provide grid operators with essential tools to maintain stability and balance in the electricity grid. They can quickly respond to changes in demand or supply, ensuring a steady voltage and frequency. This is particularly important in the context of increasing renewable energy penetration, where fluctuations in supply can pose challenges to grid stability.
- Peak Load Management: Many electricity consumers have variable energy requirements throughout the day. Electrical energy storage allows businesses and households to manage their peak loads effectively. By storing excess energy during periods of low demand and using it during peak hours, energy storage systems help to reduce strain on the grid and lower peak power consumption costs.
- Emergency Backup: In cases of emergencies or unforeseen events, having a reliable backup power source is essential. Energy storage provides the ability to quickly and efficiently supply power when the main grid fails, improving the resilience of critical infrastructure, hospitals, and other essential services.
These factors highlight the significant role that electrical energy storage plays in our energy landscape. It not only helps to optimize the utilization of renewable energy sources but also enhances the stability, reliability, and flexibility of the overall power system. As we continue to transition towards a sustainable energy future, the importance of electrical energy storage will only continue to grow.
Types of Electrical Energy Storage
There are various types of electrical energy storage technologies available, each with its own unique characteristics and applications. Here are some of the most commonly used types:
- Batteries: Batteries are perhaps the most well-known and widely used form of electrical energy storage. They store energy electrochemically and release it as needed. They come in various sizes and chemistries, including lead-acid, lithium-ion, and flow batteries. Batteries are commonly used in portable electronic devices, electric vehicles, and grid-scale applications.
- Capacitors: Capacitors store energy in an electric field created by opposing charges on two conductive plates. They can charge and discharge very quickly, making them suitable for applications that require high-power delivery. However, capacitors typically have lower energy density compared to batteries, so they are often used for short-duration energy storage and power quality improvement.
- Flywheel Energy Storage: Flywheels store energy in a rotating mechanical system. They use a spinning rotor to store energy in its kinetic form. When energy is needed, the stored rotational energy is converted back into electrical energy. Flywheels have fast response times and a long lifespan, making them suitable for applications that require frequent charge and discharge cycles.
- Pumped Hydro Storage: Pumped hydro storage is one of the oldest and most established forms of electrical energy storage. It involves using excess electricity to pump water from a lower reservoir to a higher reservoir. When electricity is needed, the stored water is released to flow downwards, passing through turbines to generate electricity.
- Compressed Air Energy Storage: Compressed air energy storage systems compress air during off-peak times and store it in underground caverns or tanks. When energy is needed, the compressed air is released, expanding and driving turbines to generate electricity. Compressed air energy storage is known for its long-duration storage capabilities and relatively low cost.
- Thermal Energy Storage: Thermal energy storage utilizes the heat or cold generated by excess electricity to store and later release energy when needed. This can be achieved through various techniques such as storing hot or cold water, using phase change materials, or utilizing thermal storage with concentrated solar power systems.
Each of these electrical energy storage technologies has its own advantages and limitations, making them suitable for specific applications and use cases. The choice of technology depends on factors such as cost, energy density, response time, lifespan, and scalability, among others. As technology continues to advance, new types of electrical energy storage are also being developed, further expanding the options available.
Understanding the different types of electrical energy storage is crucial for selecting the right technology to meet specific energy storage requirements. By utilizing a combination of storage technologies and optimizing their integration, we can create more flexible, reliable, and sustainable energy systems for the future.
Batteries
Batteries are one of the most widely used and versatile forms of electrical energy storage. They store energy in chemical form and convert it back into electrical energy when needed. Batteries have become increasingly popular due to their portability and ability to deliver energy efficiently. They are used in a wide range of applications, from powering portable electronic devices to electric vehicles and grid-scale energy storage systems.
There are several types of batteries available, each with its own unique characteristics and advantages:
- Lead-Acid Batteries: Lead-acid batteries are one of the oldest and most mature battery technologies. They consist of lead plates submerged in an electrolyte solution of sulfuric acid. Lead-acid batteries are economical and reliable, making them a popular choice for applications such as automotive starting batteries and uninterruptible power supply (UPS) systems.
- Lithium-ion Batteries: Lithium-ion batteries have gained significant popularity in recent years due to their high energy density, lightweight design, and longer lifespan. They utilize lithium ions moving between the positive and negative electrodes during charge and discharge cycles. Lithium-ion batteries are commonly used in portable electronic devices, electric vehicles, and residential or commercial energy storage systems.
- Flow Batteries: Flow batteries are a type of battery that utilizes two electrolytes separated by a membrane. The electrolytes flow through electrodes and react chemically to store and release electricity. Flow batteries offer advantages such as scalable energy capacity, long cycle life, and the ability to discharge at a constant power level. They are often used for large-scale energy storage applications and grid-level integration of renewable energy sources.
- Solid-State Batteries: Solid-state batteries are an emerging battery technology that uses solid electrolytes instead of liquid or gel electrolytes found in traditional batteries. They offer advantages such as enhanced energy density, improved safety, and faster charging capabilities. Solid-state batteries have the potential to revolutionize the battery industry by enabling safer and more efficient energy storage for various applications.
Advancements in battery technology have led to improvements in energy storage capacity, charging times, lifespan, and overall efficiency. Research and development efforts are focused on enhancing battery performance, reducing cost, and addressing concerns related to environmental impact and resource availability.
While batteries offer numerous benefits, they also have certain limitations. One of the main challenges is their limited energy storage capacity compared to other storage technologies, such as pumped hydro or compressed air energy storage. Additionally, batteries can experience performance degradation over time, affecting their overall lifespan and capacity.
Despite these challenges, batteries continue to play a pivotal role in shaping the future of electrical energy storage. Their versatility, mobility, and scalability make them suitable for a wide range of applications, from small-scale consumer electronics to large-scale grid storage, enabling us to utilize renewable energy sources more effectively and create a more sustainable energy ecosystem.
Capacitors
Capacitors are another type of electrical energy storage device that store energy in an electric field. Unlike batteries, which store energy chemically, capacitors store energy electrostatically. They consist of two conductive plates, separated by a dielectric material, which prevents direct electrical contact between the plates.
Capacitors have several characteristics that make them unique and valuable in certain applications:
- Rapid Charging and Discharging: One of the key advantages of capacitors is their ability to charge and discharge rapidly. Because there are no chemical reactions involved in the storage and discharge process, capacitors can deliver electrical energy in a very short amount of time.
- High Power Density: Capacitors have a high power density, meaning they can deliver a high amount of power relative to their size and weight. This makes them suitable for applications where a rapid burst of energy is required, such as in electric vehicles or high-power electronics.
- Long Cycle Life: Unlike batteries, which can experience degradation over time, capacitors have a virtually unlimited cycle life. They can be charged and discharged repeatedly without significant loss of performance, making them highly durable and reliable for long-term use.
- Temperature Insensitivity: Capacitors are generally not affected by temperature changes, allowing them to operate consistently in a wide range of environmental conditions. This characteristic makes them suitable for diverse applications where temperature variations can occur.
However, capacitors also have limitations that restrict their use in certain scenarios:
- Low Energy Density: Compared to batteries, capacitors generally have lower energy density. This means they cannot store as much energy per unit of volume or weight. As a result, their storage capabilities may be limited and not suitable for long-duration energy storage applications.
- Voltage Limitations: Capacitors have voltage limitations, meaning they can only store and discharge energy up to a certain voltage level. Exceeding this voltage can cause damage to the capacitor and compromise its performance.
- Self-Discharge: Capacitors have a self-discharge characteristic, meaning they gradually lose their stored charge over time. This can result in a loss of energy if the capacitor is not used promptly and can limit their effectiveness in applications that require long-term energy storage.
Capacitors find applications in a wide range of industries and technologies, including power electronics, renewable energy systems, and electronic circuits. They are commonly used for power factor correction, energy storage in regenerative braking systems, voltage regulation, and transient voltage suppression.
As technology continues to advance, researchers are exploring new materials and designs to improve the energy density and efficiency of capacitors. This includes the development of supercapacitors, also known as ultracapacitors, which offer higher energy storage capabilities compared to traditional capacitors.
In summary, capacitors provide unique advantages for specific applications that require high power delivery, rapid charging/discharging, and long cycle life. While they may not possess the high energy density of batteries, capacitors offer a complementary solution to meet specific energy storage requirements and contribute to the overall landscape of electrical energy storage.
Read more: How To Store Electricity
Flywheel Energy Storage
Flywheel energy storage is a mechanical form of energy storage that uses a rotating mass, known as a flywheel, to store and release energy. It operates on the principles of inertia and rotational kinetic energy.
Here are some key aspects of flywheel energy storage:
- Energy Storage Mechanism: Flywheels store energy by increasing the rotational speed of a spinning mass. When energy is fed into the system, the flywheel spins faster, storing the excess energy in its angular momentum. To retrieve the stored energy, the flywheel slows down, and the rotational energy is converted back into electrical energy.
- Fast Response Time: Flywheels have rapid response times and can release energy almost instantaneously. This makes them suitable for applications where a quick burst of power is needed, such as uninterruptible power supply (UPS) systems or grid stabilization during sudden changes in power demand.
- Long Cycle Life: Flywheel systems have a long cycle life and can withstand millions of charge and discharge cycles without significant performance degradation. This makes flywheel energy storage a reliable option for applications requiring frequent charge and discharge cycles.
- No Chemical Reactions: Flywheels operate without involving any chemical reactions, reducing concerns related to environmental impact, hazardous materials, and disposal considerations.
- Mechanical Design: Flywheels consist of a high-speed rotor, a containment system, and a motor-generator assembly. The rotor is made from a high-strength material such as steel or composite carbon fiber, and it spins in a low-friction magnetic or mechanical bearing system to minimize energy losses.
Flywheel energy storage offers several advantages that make it a viable option for energy storage:
- Efficiency: Flywheel systems can achieve high round-trip efficiencies, typically ranging from 85% to 95%. This means that a large portion of the energy stored in the flywheel can be successfully retrieved and used when needed.
- Scalability: Flywheel systems can be easily scaled up or down to match specific energy storage requirements. Multiple flywheels can be connected in parallel to increase the overall energy storage capacity, offering scalability to meet different power demands.
- Long Duration Storage: While flywheel systems are known for their high-power delivery capabilities, they can also provide energy storage for longer durations, depending on the system design and size. This makes them suitable for applications that require both power and energy storage.
- Durability: Flywheels are designed to be durable and require minimal maintenance. They have a long lifecycle and can withstand a wide range of environmental conditions, making them a reliable choice for energy storage.
However, there are a few limitations to consider when using flywheel energy storage:
- Self-Discharge Rate: Over time, energy stored in the flywheel can dissipate due to mechanical and aerodynamic losses. This self-discharge rate poses a challenge for long-duration storage applications.
- Physical Space Requirements: Flywheel systems typically require a significant amount of physical space due to their rotating mass and containment system. This can limit their deployment options in certain environments.
- Cost: Flywheel energy storage systems can be relatively expensive compared to other energy storage technologies, such as batteries or pumped hydro storage. However, cost reductions are being achieved through technological advancements and economies of scale.
Flywheel energy storage is well-suited for a variety of applications, including grid stabilization, frequency regulation, backup power, and integration with renewable energy sources. As research and development in flywheel technology continues, efforts are focused on improving energy storage capacity, reducing power losses, and optimizing system efficiency.
Overall, flywheel energy storage offers a promising solution for high-power, fast-response energy storage needs, contributing to a more resilient and sustainable energy future.
Consider using rechargeable batteries or a power bank to store electrical energy for portable devices. For larger scale storage, look into options such as lithium-ion batteries, pumped hydro storage, or flywheel energy storage.
Pumped Hydro Storage
Pumped hydro storage is a well-established and widely used method of electrical energy storage. It utilizes the power of gravity and water to store and release energy. Pumped hydro storage systems consist of two water reservoirs at different elevations and a reversible pump-turbine system.
Here is how pumped hydro storage works:
- Charging Mode: During times of excess electricity generation or low demand, surplus energy is used to pump water from the lower reservoir to the upper reservoir, raising its potential energy.
- Discharging Mode: When electrical energy is needed, the stored water is released from the upper reservoir and flows downhill through the reversible pump-turbine system. As the water flows through the turbine, it drives a generator to produce electricity.
Pumped hydro storage offers several advantages making it an essential component of the electrical energy storage landscape:
- Efficiency: Pumped hydro storage systems have high round-trip efficiencies, typically around 70% to 85%. This means that a significant portion of the stored energy can be recovered and used when needed.
- Long Duration Storage: Pumped hydro storage has the ability to store large amounts of energy for extended periods. It can provide hours or even days of continuous electricity supply, especially when combined with other renewable energy sources.
- Scalability: Pumped hydro storage systems can be easily scaled up or down to match specific power requirements. By adjusting the size of the two reservoirs and the power capacity of the pump-turbine system, the energy storage capacity can be customized accordingly.
- Long Operational Lifespan: Pumped hydro storage systems have a very long operational lifespan, typically spanning several decades. With proper maintenance, these systems can provide reliable storage capabilities for many years.
- Environmental Friendliness: Pumped hydro storage does not produce any greenhouse gas emissions during its operation. It also has a relatively low impact on the environment compared to other large-scale energy storage technologies.
Despite its benefits, pumped hydro storage also has a few limitations to consider:
- Geographical Limitations: Pumped hydro storage requires specific geographical features, such as a suitable location with different elevations and access to sufficient water resources. These requirements may limit the deployment of pumped hydro storage systems to certain regions.
- High Capital Costs: The upfront capital costs of building pumped hydro storage systems can be significant due to the construction of reservoirs, tunnels, and pump-turbine facilities. However, these costs can be offset by the long lifespan and economic benefits of the system over time.
- Environmental Impact: While the operation of pumped hydro storage systems is environmentally friendly, the construction process can have certain environmental impacts, such as the alteration of natural water bodies and habitats. Careful planning and mitigation measures are necessary to minimize these impacts.
Pumped hydro storage is widely used around the world, accounting for the majority of installed grid-scale energy storage capacity. It provides a reliable and flexible means of storing and delivering large amounts of electrical energy, supporting grid stability, and integrating renewable energy sources into the electricity system.
As the demand for clean and sustainable energy continues to grow, pumped hydro storage will continue to play a critical role in meeting the world’s growing energy storage needs while contributing to a more sustainable and resilient electricity grid.
Compressed Air Energy Storage
Compressed air energy storage (CAES) is a form of energy storage that utilizes pressurized air to store and release energy. It is a flexible and efficient solution that can help balance electricity supply and demand, particularly in large-scale applications. CAES systems typically consist of underground or aboveground storage vessels, compression and expansion units, and power generation components.
Here’s how compressed air energy storage works:
- Charging Mode: During periods of low electricity demand or excess generation, surplus electricity is used to power compressors that compress air and store it in underground or aboveground storage vessels.
- Discharging Mode: When electricity is needed, the compressed air is released and heated using natural gas combustion or waste heat sources. The heated air expands and drives turbines, which generate electricity. The exhaust heat can also be utilized for various purposes, improving overall system efficiency.
Compressed air energy storage offers several advantages that make it an attractive option for energy storage:
- Energy Storage Capacity: CAES systems have the potential to store large amounts of energy for extended periods, making them suitable for applications that require long-duration storage. The capacity can be increased by scaling up the size of the storage vessels.
- High Efficiency: CAES systems achieve high round-trip efficiencies, typically around 50% to 70%. By utilizing waste heat from the compression process and exhaust heat from power generation, the overall efficiency of the system can be significantly improved.
- Flexibility in Deployment: Compressed air energy storage can be deployed in various locations, both onshore and offshore. Existing underground caverns or aboveground vessels, such as depleted natural gas reservoirs or salt caverns, can be repurposed for energy storage, reducing the need for new infrastructure.
- Grid Stability: CAES systems can provide grid stability by quickly responding to fluctuations in electricity demand or supply. They can ramp up power generation by releasing the stored compressed air when demand is high and decrease power generation during periods of low demand.
- Compatibility with Renewables: Compressed air energy storage can be integrated with renewable energy sources such as wind and solar power. Excess electricity generated by renewable sources can be used to compress air and store it, allowing for better utilization of renewable energy and reducing curtailment.
However, there are a few considerations and challenges associated with compressed air energy storage:
- Geographical Limitations: Suitable geological formations or sites with underground reservoirs are required for underground compressed air storage. Aboveground storage vessels require adequate space and considerations for safety and environmental impacts.
- Heat Loss: When the compressed air is stored, some heat is lost, which reduces the overall efficiency of the system. Strategies such as thermal insulation and heat recovery techniques can help mitigate this heat loss and improve the performance of CAES systems.
- Environmental Impact: The combustion of fossil fuels, typically natural gas, in the expansion phase of CAES systems can result in carbon emissions. The utilization of carbon capture and storage technologies or the use of renewable fuels can help mitigate the environmental impact.
- Initial Investment: The initial capital cost of building a compressed air energy storage system can be significant due to the required infrastructure and equipment. However, over time, the cost can be offset by the system’s operational benefits and long-term energy storage capabilities.
Compressed air energy storage offers a flexible and reliable solution for large-scale energy storage. Its ability to store large amounts of energy, coupled with high efficiency and compatibility with renewable energy sources, makes it a valuable asset in achieving a sustainable and resilient energy future.
Thermal Energy Storage
Thermal energy storage (TES) is a method of storing and releasing energy in the form of heat or cold. It involves capturing excess thermal energy and storing it for later use, providing a flexible and efficient solution for managing energy supply and demand. Thermal energy storage systems are widely used in various industries, including buildings, industrial processes, and power generation.
Here are some key aspects of thermal energy storage:
- Energy Storage Mechanism: Thermal energy storage systems store energy by capturing and storing heat or cold generated during periods of excess energy production or low demand. This stored energy can be released and used later when there is a need for heating, cooling, or power generation.
- Types of Thermal Energy Storage: There are several types of thermal energy storage systems, including sensible heat storage, latent heat storage, and thermochemical storage. Sensible heat storage involves storing heat in a substance without any phase change, such as using heated water or rocks. Latent heat storage involves the use of materials that can absorb and release heat during phase transitions, such as the melting and solidification of phase change materials (PCMs). Thermochemical storage utilizes chemical reactions to store and release heat energy.
- Applications of Thermal Energy Storage: Thermal energy storage finds applications in various sectors. In buildings, it can be used for heating and cooling purposes, reducing the reliance on external energy sources. Industrial processes can utilize TES to store excess heat generated during high production periods and use it during periods of lower demand. Power generation facilities, especially concentrated solar power plants, employ TES to store solar energy for use during cloudy periods or at night.
Thermal energy storage offers several advantages that make it an attractive option for energy storage:
- Efficiency: Thermal energy storage systems can achieve high energy conversion efficiency, as heat can be stored and released without significant losses. This makes TES a viable option for storing and utilizing excess heat or cold efficiently.
- Cost Savings: By utilizing thermal energy storage, energy costs can be reduced by maximizing the utilization of off-peak electricity or excess thermal energy that would otherwise go to waste. This can lead to significant cost savings for industries, buildings, and power generators.
- Environmental Sustainability: Thermal energy storage systems can contribute to reducing greenhouse gas emissions by optimizing the utilization of renewable energy sources, such as solar thermal energy or waste heat recovery. By storing and utilizing this energy more efficiently, the reliance on fossil fuels can be reduced.
- Versatility: Thermal energy storage can be applied to a wide range of temperature ranges and can provide both heating and cooling capabilities. This versatility makes it suitable for various applications and sectors, allowing for greater flexibility in meeting energy demands.
However, there are certain considerations and challenges associated with thermal energy storage:
- Thermal Losses: During the storage and release of thermal energy, some heat is inevitably lost to the surrounding environment. This heat loss can reduce the overall efficiency of the system and impact the amount of stored energy available for use.
- Space and Infrastructure: Thermal energy storage systems often require dedicated infrastructure and space for storing the thermal media or phase change materials. This can sometimes limit their implementation in certain applications with limited physical space.
- Material Selection: The selection of appropriate materials for thermal energy storage is crucial to ensure efficient heat transfer and minimize thermal losses. Choosing the right materials with suitable thermal properties and phase change characteristics is essential for optimizing the performance of TES systems.
- System Design: Designing effective thermal energy storage systems requires careful consideration of factors such as heat transfer mechanisms, insulation, control systems, and integration with the existing energy infrastructure. Proper system design is crucial for maximizing the benefits of TES.
Thermal energy storage offers a reliable and sustainable solution for managing excess heat or cold and optimizing energy utilization. By capturing and storing thermal energy, it enables more efficient energy management, cost savings, and the integration of renewable energy sources. As technology continues to evolve, advancements in thermal energy storage systems are expected to further enhance their effectiveness and expand their applications.
Read more: How To Store An Electric Bike
Advancements in Electrical Energy Storage
The field of electrical energy storage is constantly evolving, driven by advancements in technology and the increasing need for efficient and sustainable energy storage solutions. These advancements are crucial in addressing the challenges associated with integrating renewable energy sources, improving grid stability, and optimizing energy utilization. Here are some notable advancements in electrical energy storage:
- Lithium-Ion Battery Technology: Lithium-ion batteries have undergone significant advancements in recent years. Improvements in battery chemistry, electrode materials, and manufacturing processes have led to higher energy densities, longer lifespans, and faster charging capabilities. These advancements have made lithium-ion batteries the preferred choice for portable electronics, electric vehicles, and grid-scale applications.
- Flow Battery Technologies: Flow batteries have gained attention due to their advantages in scalability and long-duration energy storage. Recent advancements in flow battery chemistry, such as the use of redox-active organic compounds and vanadium-based electrolytes, have improved energy storage capacity, efficiency, and cost-effectiveness. Flow batteries are now being deployed in grid-scale energy storage projects.
- Solid-State Batteries: Solid-state batteries are an emerging technology that offers enhanced safety, higher energy density, faster charging rates, and longer lifespans compared to conventional lithium-ion batteries. Researchers are exploring the use of solid electrolytes and advanced electrode materials to overcome the limitations of liquid electrolytes and improve the performance of solid-state batteries.
- Advanced Thermal Energy Storage: Advances in thermal energy storage have focused on improving heat transfer mechanisms, increasing energy storage capacity, and reducing thermal losses. Innovations in materials, such as the development of high-temperature phase change materials and novel heat exchangers, have enhanced the efficiency and cost-effectiveness of thermal energy storage systems.
- Hybrid Energy Storage Systems: Hybrid energy storage systems combine different storage technologies to leverage their unique strengths. For example, combining batteries and supercapacitors allows for high-power discharge capabilities and long-duration energy storage. These hybrid systems enhance overall performance, efficiency, and grid stability.
- Smart Grid Integration: Advancements in communication and control technologies have enabled better integration of energy storage systems with smart grids. Real-time monitoring and control systems optimize energy flow, allowing for more efficient utilization of stored energy and improved grid stability.
- Artificial Intelligence and Machine Learning: The use of artificial intelligence (AI) and machine learning techniques is increasingly being applied to energy storage systems. AI algorithms optimize energy management, predict energy demand, and enhance system performance by learning from historical data and patterns.
These advancements are driving the development of more efficient, cost-effective, and environmentally friendly electrical energy storage solutions. They are helping to address the limitations of current storage technologies and unlock new opportunities for renewable energy integration, grid stability, and sustainable energy management.
As both research and industry continue to invest in these areas, innovations in electrical energy storage technologies will play a crucial role in accelerating the transition to a low-carbon and sustainable energy future. The ongoing advancements in energy storage pave the way for increased renewable energy penetration, reduced reliance on fossil fuels, and a more resilient and reliable electricity grid.
Considerations for Efficient Energy Storage
Efficient energy storage is crucial for maximizing the benefits of electrical energy storage systems. Here are some key considerations to ensure the efficiency and effectiveness of energy storage:
- System Design: The design of an energy storage system plays a critical role in its efficiency. Proper sizing, appropriate selection of storage technology, and system configuration are essential for achieving optimal performance. The system should be designed to match the specific energy storage requirements, considering factors such as power capacity, energy capacity, and discharge duration.
- Energy Conversion Efficiency: Energy conversion efficiency measures how effectively energy is stored and retrieved from the storage system. It is important to select storage technologies with high round-trip efficiency to minimize energy losses during the charging and discharging processes. Higher energy efficiency translates to more efficient energy utilization and reduced operating costs.
- Cycle Life and Lifespan: The cycle life and lifespan of the storage system are critical considerations for long-term efficiency. It is important to choose storage technologies with a long cycle life that can withstand numerous charge and discharge cycles without significant degradation. Longer lifespan reduces the need for frequent replacements and lowers the overall cost of energy storage.
- Integration with Renewable Energy Sources: Efficient integration of energy storage with renewable energy sources is essential for maximizing their benefits. The storage system should be able to capture and store excess energy from renewable sources when they are available and release it during periods of high demand. This ensures a more reliable and stable supply of renewable energy to the grid.
- Grid Compatibility: Energy storage systems must be compatible with the existing grid infrastructure and seamlessly integrate into the electrical network. Proper voltage regulation, frequency control, and power quality management are essential for maintaining a stable and reliable grid. Additionally, the storage system’s response time in delivering stored energy should align with the grid’s requirements for rapid load balancing.
- Monitoring and Control: Real-time monitoring and control systems are essential for efficient energy storage operations. Advanced control algorithms and predictive analytics can optimize the charging and discharging cycles, ensuring that energy is stored and released at the most advantageous times. Monitoring systems provide visibility into the performance and health of the storage system, facilitating proactive maintenance and troubleshooting.
- Safety and Environmental Impact: Safety considerations are of utmost importance for energy storage systems. Implementing robust safety protocols, such as thermal management systems, fire suppression measures, and proper handling of hazardous materials, is crucial to ensure safe operation. Mitigating the environmental impact of energy storage systems, such as minimizing the use of toxic materials and properly managing end-of-life disposal, is also a key consideration for sustainable energy storage.
Considering these factors and integrating them into the planning, design, and operation of energy storage systems is essential for achieving efficient and effective energy storage. It is important to perform thorough feasibility studies, assess specific energy storage requirements, and conduct proper cost-benefit analyses to determine the most suitable storage technology and system configuration for each application.
By prioritizing efficiency and considering these critical factors, energy storage systems can optimize energy utilization, enhance renewable energy integration, improve grid stability, and contribute to a more sustainable and resilient energy future.
Conclusion
Electrical energy storage plays a vital role in our quest for a sustainable and reliable energy future. The advancements in energy storage technologies have paved the way for efficient utilization of renewable energy sources, enhanced grid stability, and improved energy management. As we continue to transition to a low-carbon economy, energy storage will become increasingly important in balancing supply and demand, maximizing the utilization of renewable resources, and optimizing grid operations.
From batteries and capacitors to pumped hydro storage and compressed air energy storage, there are various options available, each with its own advantages and limitations. Batteries provide portability and fast response times, while technologies like pumped hydro storage offer long-duration and large-capacity storage capabilities. Capacitors contribute to rapid power delivery, and compressed air energy storage ensures scalability and cost-effectiveness.
Advancements in energy storage technologies, such as lithium-ion batteries, flow batteries, and solid-state batteries, continue to improve energy density, efficiency, and lifespan. The concept of hybrid energy storage systems, combining different storage technologies, expands the flexibility and versatility of energy storage solutions. Integration of artificial intelligence and machine learning enables smarter control and optimization of energy storage systems in real-time.
Efficiency considerations, including system design, energy conversion efficiency, cycle life, and grid compatibility, are crucial for maximizing the benefits of energy storage. The integration of energy storage with renewable energy sources enhances the reliability and availability of clean power. Monitoring and control systems optimize energy flow, while safety measures and environmental considerations ensure responsible and sustainable energy storage operations.
In conclusion, electrical energy storage is at the forefront of the energy revolution. It enables us to harness renewable energy effectively, stabilize the grid, and pave the way for a more sustainable and resilient energy infrastructure. As technology continues to advance, it is important to prioritize efficient energy storage, drive innovation, and adopt comprehensive strategies to harness the full potential of energy storage for a greener and more sustainable future.
Frequently Asked Questions about How To Store Electrical Energy
Was this page helpful?
At Storables.com, we guarantee accurate and reliable information. Our content, validated by Expert Board Contributors, is crafted following stringent Editorial Policies. We're committed to providing you with well-researched, expert-backed insights for all your informational needs.
0 thoughts on “How To Store Electrical Energy”