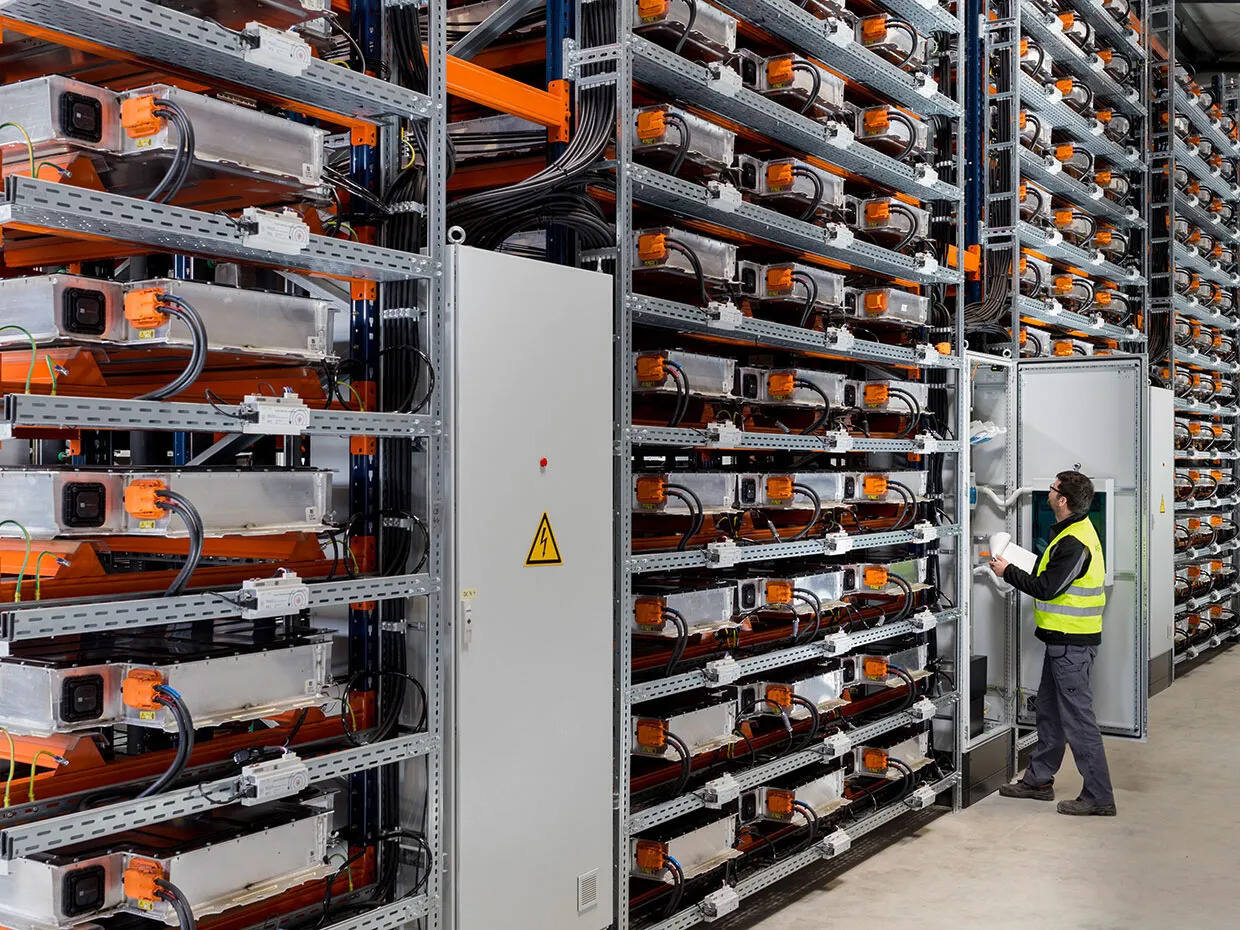
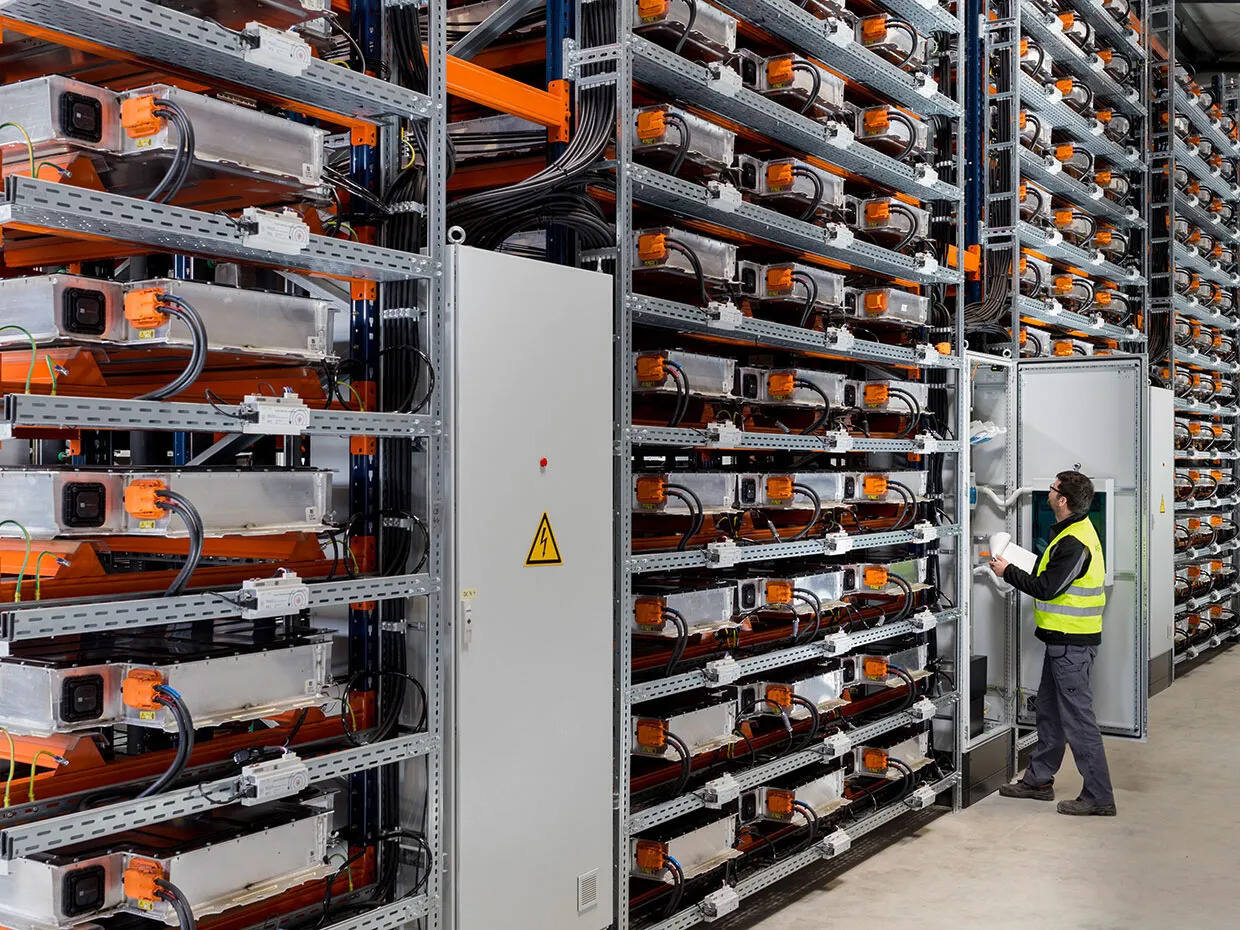
Articles
How To Store Electricity
Modified: January 8, 2024
Learn how to store electricity efficiently and safely with our informative articles. Discover the best techniques and solutions for storing renewable energy.
(Many of the links in this article redirect to a specific reviewed product. Your purchase of these products through affiliate links helps to generate commission for Storables.com, at no extra cost. Learn more)
Introduction
The demand for electricity continues to rise as society becomes increasingly reliant on electronic devices and energy-intensive activities. However, there are times when the supply of electricity fluctuates due to varying factors such as weather conditions, power plant inefficiencies, or grid malfunctions. To address this issue, the development of advanced energy storage systems has become crucial.
Energy storage systems are designed to store excess electricity during times of low demand and release it during times of high demand, thereby ensuring a stable and reliable power supply. These systems play a vital role in balancing the grid, managing intermittent renewable energy sources, and reducing the dependence on fossil fuel-based power plants.
In this article, we will explore various types of energy storage systems and their benefits. From batteries to fuel cells and beyond, these technologies offer unique advantages and have the potential to revolutionize the way electricity is stored, distributed, and utilized.
Key Takeaways:
- Diverse energy storage technologies, from batteries to fuel cells, offer solutions for grid stabilization, renewable energy integration, and peak load shifting, driving the transition to a sustainable and resilient energy future.
- Ongoing advancements in energy storage systems, such as solid-state batteries and supercapacitors, promise improved safety, higher energy density, and faster charging times, paving the way for a cleaner and more efficient global energy system.
Read more: How To Store An Electric Bike
Battery Storage Systems
Battery storage systems are one of the most commonly used and versatile forms of energy storage. They consist of rechargeable batteries that store electrical energy in chemical form and convert it back to electricity when needed. These systems range from small-scale applications, such as portable electronics, to large-scale installations that can power entire communities.
One of the key advantages of battery storage systems is their ability to store and discharge electricity quickly, making them ideal for smoothing out fluctuations in electricity supply and demand. They can rapidly respond to grid conditions, providing backup power or absorbing excess energy during times of high renewable energy generation.
Lithium-ion batteries, commonly found in smartphones and electric vehicles, are a popular choice for battery storage systems due to their high energy density and long cycle life. However, other battery chemistries, such as lead-acid and sodium-ion, are also used depending on specific requirements and cost considerations.
Battery storage systems offer several benefits, including:
- Grid Stabilization: By absorbing excess electricity during periods of low demand and discharging it when demand exceeds supply, battery storage systems help stabilize the grid and prevent blackouts.
- Renewable Integration: As renewable energy sources like solar and wind become more prevalent, battery storage systems play a crucial role in balancing the intermittent nature of these energy sources and ensuring continuous power supply.
- Peak Load Shifting: Battery storage systems can store electricity during off-peak hours when electricity costs are low and discharge it during peak periods when energy prices are higher, helping to reduce electricity bills.
- Backup Power: In areas prone to power outages, battery storage systems can provide emergency backup power to critical facilities and households, ensuring essential functions can continue unhindered.
Furthermore, advancements in battery technology, such as the development of solid-state batteries and the use of new materials, hold the promise of even higher energy densities, faster charging times, and increased safety. These improvements will further enhance the efficiency and effectiveness of battery storage systems.
Flywheel Storage Systems
Flywheel storage systems are a unique form of energy storage that utilize the principle of rotational kinetic energy to store and release electricity. These systems consist of a rotating mass, usually a flywheel, which stores energy in the form of angular momentum.
When excess electricity is available, the flywheel accelerates, storing energy in its rotational motion. When electricity is needed, the flywheel decelerates, converting its kinetic energy back into electrical energy. The speed and size of the flywheel determine the amount of energy it can store and discharge.
Flywheel storage systems offer several advantages:
- Rapid Response: Flywheels can quickly charge and discharge energy, making them ideal for applications that require fast response times, such as frequency regulation or backup power.
- High Efficiency: Due to the minimal energy loss in the mechanical system, flywheel storage systems can achieve high roundtrip efficiency, typically around 90% to 95%.
- Long Lifespan: Flywheel systems have a long lifespan, as they do not degrade over time like batteries. This makes them a durable and cost-effective option for energy storage.
- Modularity: Flywheels can be easily interconnected to create larger energy storage capacities, allowing for scalability and flexibility in meeting varying energy demands.
However, there are a few challenges associated with flywheel storage systems. One significant challenge is maintaining the stability of the rotating mass. Advanced technologies, such as magnetic bearings and vacuum enclosures, are used to minimize friction and ensure the flywheel remains stable, even at high speeds. Additionally, flywheel storage systems require a continuous power source to keep the flywheel spinning, resulting in some parasitic power loss.
Despite these challenges, flywheel storage systems offer a promising solution for various applications. They have been used in high-performance applications like grid stabilization, uninterruptible power supply (UPS) systems, and regenerative braking in transportation. Ongoing research and development in materials and design are driving improvements in energy storage capacity and overall efficiency of flywheel systems, making them an increasingly viable option in the energy storage landscape.
Pumped Storage Hydroelectricity
Pumped storage hydroelectricity is a well-established and widely used method of energy storage. It utilizes the gravitational potential energy of water to store and release electricity. This system consists of two interconnected reservoirs at different elevations and a reversible pump-turbine.
During periods of low electricity demand, when there is excess generation from power plants or renewable sources, the surplus electricity is used to pump water from the lower reservoir to the upper reservoir. This process stores energy in the form of the elevated water. When electricity demand exceeds supply, the water is released from the upper reservoir, flowing downhill through the pump-turbine to generate electricity.
Pumped storage hydroelectricity offers several significant advantages:
- High Efficiency: Pumped storage hydroelectricity exhibits high efficiency, typically around 70-80%. This is due to the ability to reuse the same water multiple times, resulting in minimal energy losses.
- Large Storage Capacity: These systems can store a substantial amount of energy, ranging from a few megawatt-hours to gigawatt-hours, making them suitable for balancing the grid and meeting peak demand requirements.
- Fast Response: Pumped storage hydroelectricity systems can quickly respond to changes in electricity demand, ramping up or down within minutes, providing grid stability and ensuring a reliable power supply.
- Long Service Life: With proper maintenance, pumped storage hydroelectricity plants can operate for several decades, making them a long-term and reliable energy storage solution.
However, there are some challenges associated with pumped storage hydroelectricity. The main challenge is the availability of suitable geographical locations with appropriate elevation differences and sufficient water resources. Finding suitable sites for new pumped storage projects can be challenging and costly. Additionally, the construction and operation of such plants can impact local ecosystems and wildlife habitats.
Despite these challenges, pumped storage hydroelectricity remains a critical component of the global energy storage infrastructure. Its ability to store large amounts of energy and provide grid stability during peak demand periods makes it an integral part of the future energy transition.
Compressed Air Energy Storage
Compressed Air Energy Storage (CAES) is a method of energy storage that utilizes compressed air to store and release electricity. This system works by compressing air and storing it in underground caverns, depleted natural gas fields, or specially designed pressurized vessels.
During periods of excess electricity, the compressed air is generated by using surplus electricity to power compressors. The compressed air is then stored until it is needed. When electricity demand exceeds supply, the pressurized air is released and expands through a turbine, generating electricity. Additional natural gas or heat sources can be used to enhance the energy efficiency of the system.
CAES offers several advantages as an energy storage solution:
- Scalability: CAES systems can be designed to store large amounts of energy, making them suitable for grid-scale applications and accommodating renewable energy sources with intermittent generation.
- Long Duration Storage: CAES can store energy for extended periods, allowing for continuous operation even during prolonged periods of low renewable energy generation or high demand.
- Low Environmental Impact: CAES systems do not emit greenhouse gases during operation, making them a relatively environmentally friendly energy storage option.
- Recovery of Waste Heat: Some CAES systems can recover and utilize the waste heat generated during compression and expansion processes for other purposes, increasing overall system efficiency.
However, there are a few challenges associated with CAES. One challenge is the energy efficiency of the system, as there can be energy losses during the compression and expansion processes. Additionally, finding suitable geological formations for underground storage or constructing above-ground storage vessels can be challenging and site-specific.
Ongoing research and development efforts are focused on improving the efficiency and reducing the costs associated with CAES technology. Advancements in materials, compression technologies, and storage methods are expected to make CAES an increasingly viable and cost-effective option for large-scale energy storage in the future.
Read more: How To Store Electric Guitar
Thermal Energy Storage
Thermal energy storage is a method of storing and utilizing heat energy for various applications. This technology allows excess energy to be stored as heat and released when needed, offering a flexible and efficient approach to energy storage.
There are different types of thermal energy storage systems, including sensible heat storage, latent heat storage, and thermochemical heat storage.
Sensible Heat Storage: Sensible heat storage involves storing thermal energy by increasing or decreasing the temperature of a material without undergoing a phase change. Common materials used for sensible heat storage include water, rocks, and molten salts. These materials have high heat capacities, allowing them to store and release large amounts of heat energy.
Latent Heat Storage: Latent heat storage involves storing thermal energy by utilizing the phase change of a material, such as melting or freezing. Materials with high latent heat of fusion, like certain salts and paraffins, can store large amounts of energy during phase transitions. This energy can be released when the material solidifies or melts.
Thermochemical Heat Storage: Thermochemical heat storage relies on reversible chemical reactions to store and release heat energy. These systems use materials that can undergo chemical reactions, absorbing or releasing heat in the process. When heat is supplied, the material undergoes a chemical reaction that stores heat energy. When heat is needed, the material undergoes a reverse reaction, releasing the stored heat.
Thermal energy storage offers several advantages:
- Flexible Applications: Thermal energy can be stored and utilized for various applications, such as space heating and cooling, industrial processes, and power generation.
- Efficient Energy Conversion: Thermal energy storage systems can achieve high energy conversion efficiencies, as they operate on the principle of heat transfer rather than electrical conversions.
- Long Duration Storage: Thermal energy can be stored for extended periods, allowing for continuous operation even during periods of low energy generation or high demand.
- Economic Viability: Depending on the specific application, thermal energy storage systems can offer cost-effective solutions by reducing peak electricity demand and optimizing energy utilization.
Challenges associated with thermal energy storage include the need for appropriate insulation to minimize heat losses, selecting suitable materials with high energy storage capabilities, and ensuring system reliability and safety.
Ongoing advancements in materials science, heat transfer technologies, and system design are driving the development of more efficient and cost-effective thermal energy storage systems. These systems have the potential to play a significant role in balancing energy supply and demand, improving energy utilization, and reducing greenhouse gas emissions in various sectors.
Consider using rechargeable batteries to store electricity. Make sure to properly maintain and store them in a cool, dry place to prolong their lifespan.
Capacitor Storage Systems
Capacitor storage systems are a type of energy storage that utilize capacitors to store and release electrical energy. Unlike batteries, which store energy in chemical form, capacitors store energy in an electric field between two charged conductive plates.
Capacitors consist of two electrodes separated by a dielectric material, which prevents direct electrical conduction. When a voltage is applied, the electric field is established, storing energy in the form of electrostatic potential. Capacitor storage systems can store and deliver energy rapidly, making them suitable for applications that require high-power bursts or short-duration energy release.
Capacitor storage systems offer several advantages:
- Rapid Charging and Discharging: Capacitors can charge and discharge energy at very high rates, allowing for quick response times and high-power output.
- Long Cycle Life: Capacitors have long cycle lives compared to batteries, making them a durable and reliable energy storage solution.
- Efficiency: Capacitors have low internal resistance, resulting in minimal energy losses during charge and discharge cycles, leading to high efficiency.
- Compact Size: Capacitors can store a large amount of energy in a relatively small physical footprint, making them suitable for space-constrained applications.
However, there are a few challenges associated with capacitor storage systems. One limitation is their relatively lower energy density compared to batteries. This means that capacitors can store less energy per unit volume or weight, making them less suitable for applications requiring long-term energy storage. Additionally, the voltage drop across a capacitor is proportional to the energy stored, which can impact the overall system voltage stability.
Capacitor storage systems are commonly used in applications such as regenerative braking systems in electric vehicles, smoothing out power fluctuations in renewable energy systems, and providing short-duration power backup in electronic devices. Ongoing research and development are focused on improving the energy density of capacitors, developing innovative materials, and exploring new capacitor designs to enhance their energy storage capabilities.
Overall, capacitor storage systems offer a unique set of advantages, making them a viable option for specific applications that require high-power output and rapid energy delivery.
Supercapacitors
Supercapacitors, also known as ultracapacitors or electrochemical capacitors, are energy storage devices that bridge the gap between conventional capacitors and batteries. They store energy electrostatically, similar to standard capacitors, but with significantly higher capacitance and energy density.
Supercapacitors store energy through the separation of positive and negative charges on the surface of specially designed electrodes. This separation creates an electrical double layer, also known as an electric double-layer capacitor (EDLC). The high surface area and porous nature of the electrodes allow for greater charge storage and rapid charge/discharge capabilities.
Compared to traditional capacitors, supercapacitors offer several advantages:
- Rapid Charging and Discharging: One of the key advantages of supercapacitors is their ability to charge and discharge energy rapidly, often within seconds or minutes. This makes them ideal for applications that require quick bursts of power.
- Long Cycle Life: Supercapacitors can endure hundreds of thousands to millions of charge/discharge cycles without significant performance degradation. Their long lifespan makes them a durable and reliable energy storage option.
- High Power Density: Supercapacitors have a high power density, allowing them to deliver high amounts of power quickly. This makes them suitable for applications that require high-power output.
- Wide Operating Temperature Range: Supercapacitors can operate in extreme temperatures, ranging from very cold to very hot conditions, without significant degradation in performance.
- Safety: Supercapacitors are generally considered safe because they do not contain toxic or flammable materials like some batteries do. They also have a lower risk of thermal runaway or explosion.
While supercapacitors offer unique advantages, they also have some limitations. One major limitation is their relatively lower energy density compared to batteries. This means that they can store less energy per unit volume or weight. Additionally, supercapacitors have higher self-discharge rates compared to batteries, which can result in energy loss over time.
Supercapacitors find applications where their specific advantages shine, such as regenerative braking in vehicles, providing peak power for electric grid stabilization, rapid energy storage and release in renewable energy systems, and backup power in electronic devices.
Ongoing research and development initiatives are focused on increasing the energy density of supercapacitors, exploring new electrode materials, and improving their overall performance. The combination of high-power output, fast charging, and long cycle life makes supercapacitors an exciting energy storage technology with potential for various future applications.
Solid-State Batteries
Solid-state batteries are a new and promising technology that aims to overcome the limitations of traditional lithium-ion batteries by using solid-state electrolytes instead of liquid electrolytes. These batteries offer several potential advantages, including improved safety, higher energy density, longer lifespan, and faster charging times.
In traditional lithium-ion batteries, a liquid electrolyte facilitates the movement of ions between the positive and negative electrodes. However, this liquid electrolyte can be flammable and prone to leakage, leading to safety concerns. Solid-state batteries, on the other hand, use solid electrolytes that are stable, non-flammable, and less susceptible to leakage, resulting in enhanced safety features.
Additionally, solid-state batteries have the potential for higher energy density, which means they can store more energy in the same volume or weight compared to traditional lithium-ion batteries. This increased energy density allows for longer-lasting devices and greater driving ranges for electric vehicles.
Another advantage of solid-state batteries is their potential for longer lifespan. The solid electrolytes used in these batteries are less prone to degradation and wear and tear compared to liquid electrolytes, allowing for more charge and discharge cycles before capacity starts to diminish. This extended lifespan makes solid-state batteries suitable for applications where durability and longevity are essential, such as in electric vehicles.
Faster charging times are also one of the anticipated benefits of solid-state batteries. Solid electrolytes enable faster ion diffusion compared to liquid electrolytes, resulting in reduced charging times. This feature is especially important for the widespread adoption of electric vehicles, as it addresses one of the main concerns – the time it takes to recharge the vehicle’s battery.
While solid-state batteries show great promise, there are practical challenges in their development and commercialization. One significant challenge is improving the performance and stability of solid electrolytes to enable high ionic conductivity at room temperature. Scientists are actively researching and developing new materials to overcome this challenge and optimize the performance of solid-state batteries.
Overall, solid-state batteries offer the potential to revolutionize energy storage, providing safer and more efficient power solutions. As research and development efforts continue to progress, we can expect to see solid-state batteries playing a crucial role in various applications, including electric vehicles, portable electronics, and renewable energy systems.
Read more: How To Store An Electric Guitar
Fuel Cells
Fuel cells are electrochemical devices that convert chemical energy directly into electrical energy through a reaction between a fuel and an oxidant. They offer a clean and efficient way to generate electricity, with various types of fuel cells designed to operate on different fuels.
One of the most common types of fuel cells is the hydrogen fuel cell. In a hydrogen fuel cell, hydrogen gas is supplied to the anode (negative electrode), while oxygen or air is supplied to the cathode (positive electrode). The electrolyte allows the flow of ions while preventing the mixing of the hydrogen and oxygen gases. As hydrogen molecules are split into protons and electrons, the protons move through the electrolyte and combine with oxygen at the cathode, while the electrons flow through an external circuit, generating an electric current.
Fuel cells offer several advantages over conventional combustion-based power generation:
- High Efficiency: Fuel cells can achieve higher energy conversion efficiencies compared to traditional combustion methods, with some fuel cells reaching over 60% efficiency in converting fuel to electricity.
- Clean Energy Generation: Fuel cells produce electricity without harmful emissions, as the byproducts are typically water vapor and, depending on the fuel used, trace amounts of carbon dioxide. They contribute to reduced greenhouse gas emissions and air pollution.
- Quiet Operation: Fuel cells operate silently, as there are no moving parts involved in the electricity generation process. This makes them suitable for noise-sensitive environments.
- Fuel Flexibility: Different types of fuel cells can operate on various fuels, such as hydrogen, natural gas, methanol, and even biogas. This makes fuel cells adaptable to different energy sources and reduces dependence on a single fuel type.
- Modularity: Fuel cells can be easily scaled up or down, allowing for flexible deployment in a range of applications, from small portable devices to large-scale power generation.
However, there are challenges associated with fuel cells, particularly in terms of infrastructure and cost. The availability and distribution of hydrogen infrastructure, for example, present obstacles for widespread adoption. Additionally, the cost of fuel cell systems, including the production and storage of hydrogen, remains relatively high compared to conventional power generation technologies.
Nonetheless, ongoing research and development efforts are focused on improving the performance, durability, and cost-effectiveness of fuel cells. As advances in materials, manufacturing processes, and infrastructure continue, fuel cells have the potential to become a key player in clean and efficient energy generation for a wide range of applications, including transportation, stationary power generation, and portable electronics.
Conclusion
Energy storage systems play a vital role in the modern world, enabling the efficient and reliable use of electricity. From batteries to flywheels, and from compressed air to thermal energy storage, a diverse range of technologies has emerged to meet the growing demand for energy storage solutions.
Battery storage systems, with their versatility and rapid response capabilities, offer effective grid stabilization, renewable energy integration, and peak load shifting. Flywheel storage systems provide high-power output and fast response times, making them suitable for applications that require immediate bursts of energy. Pumped storage hydroelectricity, with its large-scale storage capacity, contributes significantly to grid stability and peak demand management. Compressed air energy storage offers scalability and long-duration storage capabilities, utilizing the energy potential of pressurized air. Thermal energy storage systems store energy in the form of heat, finding applications in climate control, industrial processes, and power generation. Capacitor storage systems deliver high-power output and rapid energy delivery, making them valuable for power-intensive applications. Supercapacitors showcase rapid charging and discharging capabilities, making them ideal for applications requiring quick bursts of power. Solid-state batteries offer improved safety, higher energy density, longer lifespan, and faster charging times, presenting promising solutions for future energy storage needs. Lastly, fuel cells provide clean and efficient electricity generation, with the potential to utilize various fuels and reduce dependence on fossil fuels.
While each energy storage system has its strengths and limitations, ongoing research and development efforts are continuously improving these technologies. Advancements in materials science, engineering, and manufacturing processes are driving the efficiency, energy density, and cost-effectiveness of storage systems. As a result, the future of energy storage looks promising, with increased affordability, scalability, and sustainability.
From enhancing renewable energy integration to ensuring reliable power supply in critical applications, energy storage systems play a crucial role in the transition towards a more sustainable and resilient energy future. By harnessing the potential of these diverse storage technologies, we can create a cleaner, more efficient, and more secure global energy system.
Frequently Asked Questions about How To Store Electricity
Was this page helpful?
At Storables.com, we guarantee accurate and reliable information. Our content, validated by Expert Board Contributors, is crafted following stringent Editorial Policies. We're committed to providing you with well-researched, expert-backed insights for all your informational needs.
0 thoughts on “How To Store Electricity”