Home>Articles>What Causes Rotational Motion In An Electric Motor.
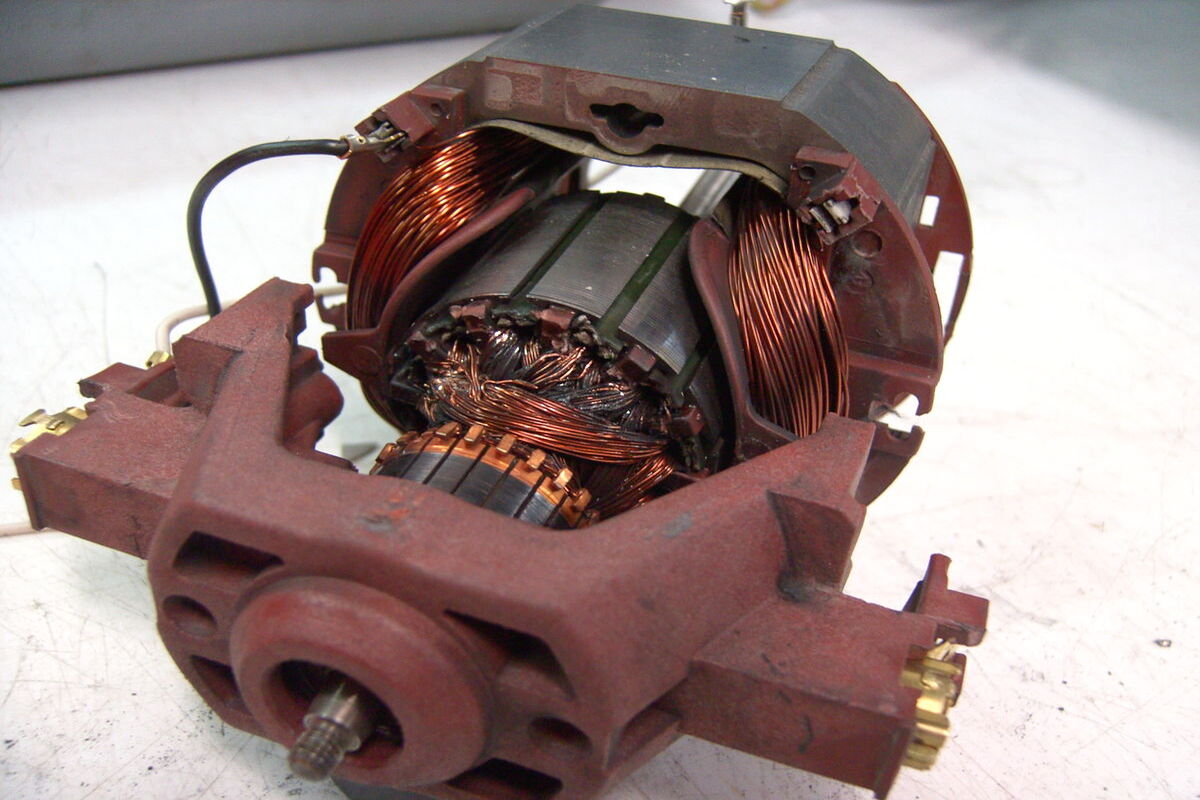
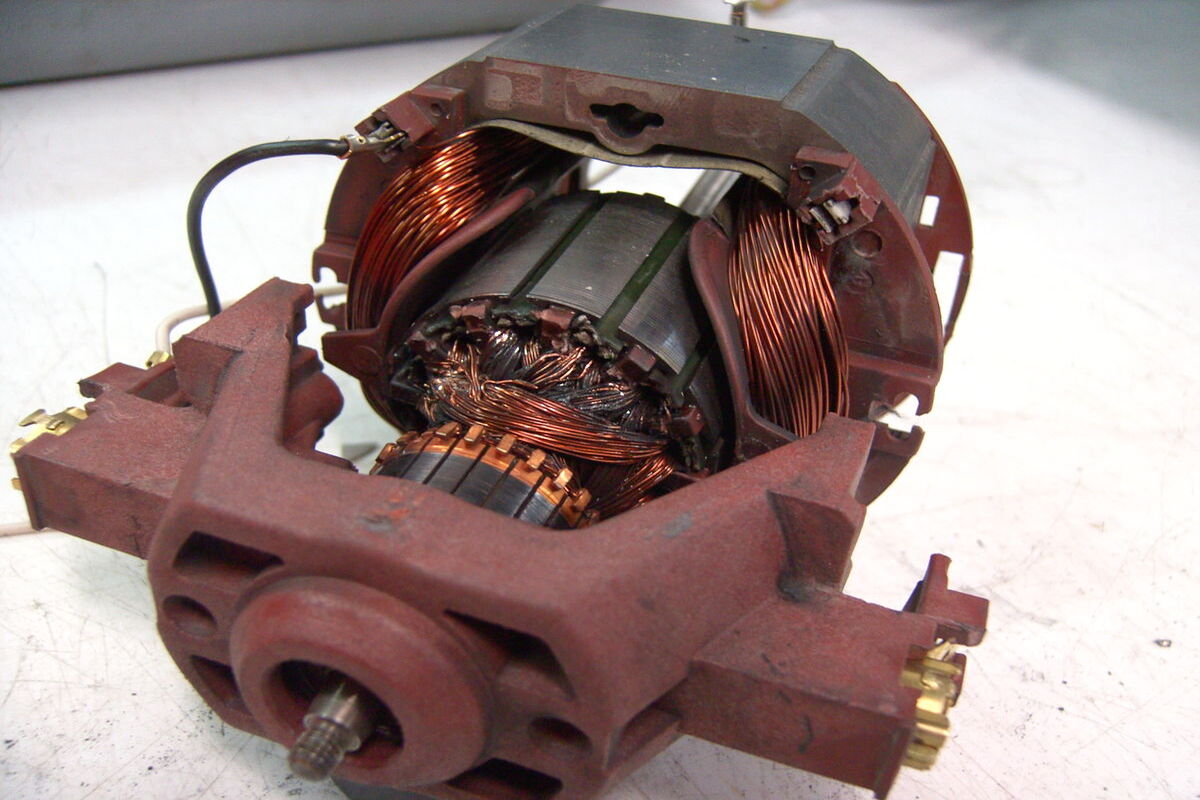
Articles
What Causes Rotational Motion In An Electric Motor.
Modified: January 7, 2024
Discover the key factors that contribute to the rotational motion in an electric motor. Read our informative articles to explore this fascinating topic in depth.
(Many of the links in this article redirect to a specific reviewed product. Your purchase of these products through affiliate links helps to generate commission for Storables.com, at no extra cost. Learn more)
Introduction
Electric motors play a crucial role in various industries and technologies, powering everything from household appliances to industrial machinery. These motors rely on the fascinating phenomenon of rotational motion to convert electrical energy into mechanical energy, enabling them to perform a wide range of tasks.
In this article, we will delve into the world of electric motors and explore what causes rotational motion in these devices. We will discuss the components of an electric motor, the principles of electromagnetism, and how rotational motion is generated and harnessed. By understanding the mechanics behind rotational motion in electric motors, we can gain a deeper appreciation for their functionality and efficiency.
So, let’s dive into the fascinating world of electric motors and uncover the secrets of rotational motion!
Key Takeaways:
- Electric motors harness rotational motion through the interaction of magnetic fields, converting electrical energy into mechanical energy. Understanding the factors affecting rotational motion is crucial for optimizing motor performance and efficiency.
- The principles of electromagnetism and the components of an electric motor work in harmony to generate rotational motion, driving innovation and powering various devices and machinery. Appreciating the role of rotational motion enhances our understanding of these remarkable devices.
Read more: What Causes An Electric Motor To Overheat
Understanding Rotational Motion
Rotational motion is the movement of an object around an axis or a fixed point. Unlike linear motion, which involves moving in a straight line, rotational motion occurs when an object rotates or spins on its axis. This type of motion is inherent in many mechanical systems, including electric motors.
When an object undergoes rotational motion, it experiences certain key properties, such as angular velocity, angular acceleration, and torque. Angular velocity refers to the rate at which the object rotates, measured in radians per second. Angular acceleration, on the other hand, describes how quickly the angular velocity of the object changes over time.
To better understand rotational motion, let’s consider an everyday example: a spinning top. When you spin a top on a flat surface, it rotates around its central point, maintaining a stable spin for a certain period. The force you apply initially determines the angular velocity of the top, while subsequent forces or interactions influence its angular acceleration.
Rotational motion follows certain principles, such as the conservation of angular momentum and Newton’s second law for rotational motion. The conservation of angular momentum states that the total angular momentum of a closed system remains constant unless acted upon by an external torque. Newton’s second law for rotational motion relates torque, moment of inertia, and angular acceleration, stating that the torque exerted on an object is equal to the moment of inertia times the angular acceleration.
Overall, understanding the principles and properties of rotational motion is crucial in comprehending how electric motors operate. With this foundational knowledge, we can now explore the components and mechanisms behind the generation of rotational motion in an electric motor.
Components of an Electric Motor
An electric motor comprises several essential components that work together to produce rotational motion. Understanding these components is crucial in comprehending how an electric motor functions. Let’s take a closer look at each of them:
- Stator: The stator is the stationary part of the electric motor. It consists of a core made of ferromagnetic material, usually laminated to reduce eddy current losses. The stator contains several coils of wire arranged in specific patterns, known as windings. These windings produce a magnetic field when an electric current flows through them.
- Rotor: The rotor is the rotating part of the electric motor. It is usually made of a series of laminated iron cores with conductive bars or coils, known as rotor windings. The rotor is placed inside the stator and is free to rotate around an axis.
- Shaft: The shaft is a cylindrical component that connects the rotor to external mechanical systems. It transfers the rotational motion generated by the motor to the desired output device or load.
- Bearings: Bearings are essential in an electric motor as they support the rotor and allow it to rotate smoothly. They reduce friction and ensure proper alignment, minimizing wear and tear on the motor components.
- Commutator: In certain types of electric motors, such as DC motors, a commutator is used to switch the direction of current flow in the rotor windings. It consists of a set of copper segments insulated from each other and connected to the rotor windings. The commutator ensures a continuous connection with the electrical power source, enabling the rotor to rotate continuously.
- Brushes: Brushes are conductive elements that make direct contact with the commutator. They deliver the electrical current to the rotor windings through the commutator, allowing the motor to function.
These components work in tandem to generate the necessary magnetic fields and electrical currents, resulting in the production of rotational motion in an electric motor. The stator windings create a magnetic field that interacts with the rotor windings, causing the rotor to rotate. The rotation of the rotor, in turn, drives the mechanical output of the motor.
Now that we have a clear understanding of the components of an electric motor, let’s explore the principles of electromagnetism and how they contribute to the generation of rotational motion.
Principles of Electromagnetism
Electromagnetism is the branch of physics that deals with the relationship between electricity and magnetism. Understanding the principles of electromagnetism is crucial in comprehending how these forces interact to generate rotational motion in an electric motor. Let’s explore some key principles:
- Magnetic Fields: Magnetic fields are areas around magnets or current-carrying conductors where magnetic forces are exerted. In the context of an electric motor, magnetic fields play a vital role in generating and controlling rotational motion. They are created by the flow of electric current through the stator windings.
- Electromagnetic Induction: Electromagnetic induction is the process in which a changing magnetic field induces an electromotive force (EMF) or voltage in a conductor. In an electric motor, this principle is utilized to generate the necessary electrical currents that drive the motor. As the rotor rotates, it cuts through the magnetic field generated by the stator windings, resulting in the induction of voltage in the rotor windings.
- Faraday’s Law: Faraday’s law of electromagnetic induction states that the magnitude of the induced EMF or voltage is directly proportional to the rate of change of magnetic field flux through a conductor. This principle explains how the rotation of the rotor and the changing magnetic field lead to the generation of electrical currents in the rotor windings.
- Lenz’s Law: Lenz’s law is a fundamental concept in electromagnetism that describes the direction of the induced current in a conductor. According to Lenz’s law, the direction of the induced current is such that it opposes the change in the magnetic field that produced it. This law ensures that the rotation of the rotor in an electric motor is stable and consistent.
These principles of electromagnetism form the basis for the operation of an electric motor. By manipulating magnetic fields and utilizing electromagnetic induction, electric motors can convert electrical energy into mechanical energy, resulting in rotational motion.
Now that we have a solid understanding of the principles of electromagnetism, let’s explore how magnetic fields are generated within an electric motor.
Magnetic Field Generation
In an electric motor, magnetic fields are crucial for the generation of rotational motion. These magnetic fields are produced by the flow of electric current through the stator windings. Let’s explore how magnetic fields are generated within an electric motor:
The stator windings consist of coiled wires that are electrically connected and arranged in specific patterns. When an electric current flows through these windings, it creates a magnetic field around the wires. This magnetic field follows the right-hand rule, where the direction of the magnetic field is determined by the direction of the current flow.
The magnetic field generated by the stator windings is known as the stator field. It remains stationary, as the stator is the fixed component of the motor. The strength and direction of the stator field can be controlled by varying the magnitude and direction of the current flowing through the stator windings.
In certain types of electric motors, such as induction motors, the magnetic field is produced using alternating current (AC). The alternating current in the stator windings creates a constantly changing magnetic field, which induces voltage in the rotor windings and initiates rotation.
In other types of motors, such as direct current (DC) motors, the magnetic field is produced using direct current. Here, the stator windings are connected to a power source, and the resulting steady-state current flow generates a constant magnetic field.
In both AC and DC motors, the strength of the magnetic field can be further enhanced by using magnetic materials, such as permanent magnets or magnetic cores, in the stator or rotor. These materials increase the magnetic flux density and allow for stronger interaction between the stator and rotor magnetic fields, resulting in more efficient and powerful rotational motion.
The generation of magnetic fields within an electric motor is a critical step in the overall process of rotational motion. The interaction between these magnetic fields and the rotor windings is what enables the conversion of electrical energy to mechanical energy, driving the rotation of the motor.
Now that we understand how magnetic fields are generated, let’s explore the interaction between these fields within an electric motor to facilitate rotational motion.
The rotational motion in an electric motor is caused by the interaction between the magnetic field and the current flowing through the wire coils, known as the Lorentz force. This force creates a torque that causes the motor to rotate.
Read more: What Causes An Electric Motor To Lose Power
Interaction of Magnetic Fields
The interaction of magnetic fields is a fundamental aspect of how an electric motor generates rotational motion. Understanding this interaction is key to comprehending the intricate workings of the motor. Let’s explore how magnetic fields interact within an electric motor:
When an electric current flows through the stator windings, it generates a magnetic field around the stator. Simultaneously, the rotor’s magnetic field is initiated by the rotor windings or permanent magnets. The interaction of these magnetic fields is what drives the rotation of the motor.
In an AC motor, where the stator field is constantly changing due to the alternating current, the magnetic fields of the stator and rotor continuously interact. As the stator field alternates, it induces a magnetic field in the rotor. This induced magnetic field created in the rotor attracts or repels the stator field depending on the polarity, causing the rotor to rotate in the direction of the rotating magnetic field.
In a DC motor, the interaction between the stator and rotor magnetic fields is facilitated by the commutator and brushes. The commutator ensures that the orientation of the current flowing through the rotor windings is maintained, allowing for a consistent magnetic field. The brushes, in contact with the commutator, ensure a continuous flow of current to the rotor windings, enabling the interaction with the stator field. The resulting magnetic forces cause the rotor to rotate.
The interaction of the magnetic fields is governed by the principles of electromagnetic induction. As the rotor rotates, it continuously cuts through the changing magnetic field produced by the stator. This cutting of magnetic flux generates an electromotive force (EMF) in the rotor windings, inducing currents. These currents, referred to as rotor currents, interact with the stator field, creating electromagnetic forces that contribute to the rotational motion.
In addition to electromagnetic forces, torque plays a crucial role in the interaction of magnetic fields. Torque is the twisting force that produces rotation. In an electric motor, the interaction of magnetic fields generates torque, which drives the rotation of the motor. The amount of torque produced depends on various factors, including the strength of the magnetic fields, the number of turns in the windings, and the distance between the stator and rotor.
By understanding and harnessing the interaction of magnetic fields, electric motors can efficiently convert electrical energy into mechanical energy, driving the rotation and functionality of various devices and machinery.
Now that we have explored the interaction of magnetic fields, let’s delve into how rotational motion is facilitated within an electric motor.
Conversion of Electrical Energy to Mechanical Energy
The conversion of electrical energy to mechanical energy lies at the heart of how an electric motor operates. This process involves harnessing the interaction of magnetic fields to produce rotation. Let’s explore how electrical energy is converted into mechanical energy within an electric motor:
1. Power Supply: To initiate the conversion process, the electric motor is connected to a power supply. The power supply provides the necessary electrical energy in the form of voltage and current to the motor.
2. Flow of Electrical Current: The electrical current flows through the stator windings, which are positioned around the rotor. This current creates a magnetic field, known as the stator field, due to the principles of electromagnetism.
3. Interplay of Magnetic Fields: The stator field interacts with the rotor’s magnetic field, resulting in a rotational force or torque. This interaction is facilitated by the rotation of the rotor, which cuts through the changing magnetic field produced by the stator.
4. Electromagnetic Induction: As the rotor rotates, it induces an electromotive force (EMF) or voltage in the rotor windings. This electromagnetic induction occurs due to the changing magnetic field cutting through the rotor windings, following Faraday’s law of electromagnetic induction.
5. Rotor Motion: The induced voltage in the rotor windings produces currents, referred to as rotor currents. These rotor currents interact with the stator field, resulting in electromagnetic forces that contribute to the rotational motion of the rotor.
6. Mechanical Output: The rotation of the rotor is transferred to the external mechanical system through the shaft. The mechanical output can be used to power various devices and perform a wide range of tasks, depending on the specific application of the electric motor.
Throughout this process, electrical energy is transformed into mechanical energy. The conversion is achieved by exploiting the principles of electromagnetism and the interaction of magnetic fields. The continuous supply of electrical energy from the power source ensures that the rotor keeps rotating, allowing for stable and consistent mechanical output.
It’s important to note that the efficiency of the conversion process varies depending on the type of electric motor and its design. Factors such as the quality of the magnetic materials, the winding configuration, and the motor’s overall efficiency can affect the effectiveness of the conversion.
By converting electrical energy to mechanical energy, electric motors have become indispensable in various industries and applications, powering everything from household appliances to large industrial machinery. Their ability to efficiently convert electrical energy to rotational motion has revolutionized modern technology and continues to drive innovation.
Now that we have explored the conversion of electrical energy to mechanical energy, let’s discuss the factors that can affect the rotational motion in an electric motor.
Role of Rotational Motion in an Electric Motor
Rotational motion plays a crucial role in the operation and functionality of an electric motor. It is the primary outcome of the motor’s conversion of electrical energy into mechanical energy. Let’s take a closer look at the role of rotational motion in an electric motor:
1. Generating Mechanical Power: Rotational motion is responsible for generating mechanical power within the electric motor. As the rotor spins, the mechanical output is transferred through the shaft to external systems and devices. This mechanical power is used to perform various tasks, such as driving machinery, spinning a fan, or powering a vehicle.
2. Moving Loads: The rotational motion produced by an electric motor allows it to move loads and perform work. The torque generated by the interaction of magnetic fields enables the motor to overcome resistance and move objects, making it essential in transportation systems, manufacturing processes, and countless other applications.
3. Enabling Control and Speed Regulation: The ability to control and regulate the rotational motion of an electric motor is crucial in many applications. By adjusting the magnitude and direction of the electric current flowing through the stator windings, the speed and direction of the motor can be controlled. This control allows for precise and efficient operation in a wide range of scenarios.
4. Converting Energy Efficiently: Rotational motion facilitates the efficient conversion of electrical energy to mechanical energy within the motor. Through the interaction of magnetic fields and the generation of torque, the electric motor maximizes the conversion efficiency, ensuring that minimal energy is wasted in the process. This makes electric motors a sustainable and cost-effective choice for various industries.
5. Facilitating Versatility: The ability to convert electrical energy to rotational motion gives electric motors tremendous versatility. They can be designed and configured to meet specific application requirements, such as different power ratings, speeds, and torque outputs. This flexibility allows electric motors to be used in a wide range of applications, from small household appliances to large industrial machinery, providing the necessary rotational motion for optimal performance.
Overall, rotational motion is the driving force behind an electric motor’s functionality. It enables the conversion of electrical energy to mechanical energy, facilitates the movement of loads, and allows for precise control and regulation of speed. By harnessing the power of rotational motion, electric motors have revolutionized various industries and contributed to the advancement of modern technology.
Now, let’s explore the factors that can affect the rotational motion in an electric motor.
Factors Affecting Rotational Motion in an Electric Motor
Several factors can influence the rotational motion of an electric motor. Understanding these factors is essential for optimizing the performance and efficiency of the motor. Let’s explore some key factors that can affect rotational motion:
1. Supply Voltage: The voltage supplied to the electric motor plays a significant role in determining its rotational motion. Higher voltages tend to result in faster rotation, while lower voltages can lead to slower rotation. It is important to ensure that the motor is operated within the recommended voltage range specified by the manufacturer to maintain optimum performance.
2. Load: The load placed on the motor can affect its rotational motion. Heavier loads typically require more torque to overcome resistance and move, leading to a slower rotational speed. Conversely, lighter loads may allow for faster rotation. Understanding the load requirements of the motor and ensuring it is appropriately matched to the motor’s capabilities are necessary for optimal performance.
3. Motor Design: The design and construction of the motor can also impact its rotational motion. Factors such as the size and shape of the rotor and stator, the type and arrangement of the windings, and the presence of additional components like gears or pulleys can influence rotational speed and torque. A well-designed motor with optimized components can contribute to smoother and more efficient rotational motion.
4. Friction and Bearing Efficiency: Friction within the motor can cause resistance and affect the rotational motion. Efficient bearings minimize friction and allow for smoother rotation. Regular maintenance, lubrication, and ensuring the proper alignment of bearings can help minimize friction and optimize rotational motion.
5. Temperature: Temperature can affect the efficiency and performance of an electric motor. High temperatures can lead to increased resistance and electrical losses, which may impact the motor’s ability to generate rotational motion effectively. Adequate cooling mechanisms and monitoring the motor’s temperature can help maintain optimal performance and prevent overheating.
6. Efficiency of Electrical Connections: The efficiency of electrical connections, such as terminals, wires, and connectors, can impact the flow of electrical current and, consequently, the generation of rotational motion. Loose or faulty connections can result in voltage drops, increased resistance, and reduced motor performance. Regular inspection and maintenance of electrical connections are necessary to ensure optimal rotational motion.
7. Motor Control: The control mechanisms employed to regulate the motor’s speed and direction can affect its rotational motion. Accurate and responsive control systems can enable precise adjustments to the motor’s parameters and enhance its performance. Proper installation, calibration, and tuning of control systems are crucial to achieving desired rotational motion.
By considering and optimizing these factors, it is possible to enhance the rotational motion of an electric motor, ensuring efficient and reliable performance in various applications.
Now that we have explored the factors that affect rotational motion, let’s conclude our discussion.
Read more: How To Reverse An Electric Motor Rotation
Conclusion
Electric motors are remarkable devices that rely on the science of rotational motion to convert electrical energy into mechanical energy. Understanding the principles and components of an electric motor allows us to appreciate how these motors power a wide range of applications, from everyday household appliances to complex industrial machinery.
Rotational motion, generated through the interaction of magnetic fields, is the driving force behind an electric motor’s functionality. By harnessing the principles of electromagnetism, electric motors are capable of converting electrical energy into the rotational motion required to perform various tasks. As the motor’s components, such as the stator, rotor, shaft, commutator, and brushes, work in cohesion, they produce the necessary magnetic fields and facilitate the conversion process.
Throughout this article, we have explored the importance of understanding rotational motion in an electric motor. We have examined key factors, such as supply voltage, load, motor design, friction, temperature, electrical connections, and motor control, that can influence the motor’s rotational motion. By considering and optimizing these factors, we can enhance the performance and efficiency of the electric motor.
Electric motors have revolutionized industries and technologies, providing reliable and efficient means of powering various devices and machinery. Their ability to convert electrical energy into rotational motion has made them indispensable in modern society.
In conclusion, understanding the principles and components of an electric motor and the role of rotational motion within it allows us to appreciate the complexity and ingenuity behind these devices. By optimizing the factors that affect rotational motion, we can maximize the performance and efficiency of electric motors, driving innovation and advancing technology in countless applications.
So, the next time you turn on a device powered by an electric motor or witness the effortless rotation of a mechanical system, take a moment to appreciate the intricate mechanics and the role of rotational motion that make it all possible.
Frequently Asked Questions about What Causes Rotational Motion In An Electric Motor.
Was this page helpful?
At Storables.com, we guarantee accurate and reliable information. Our content, validated by Expert Board Contributors, is crafted following stringent Editorial Policies. We're committed to providing you with well-researched, expert-backed insights for all your informational needs.
0 thoughts on “What Causes Rotational Motion In An Electric Motor.”